Expedition to Southern Lands
This post is the second part of a day-by-day timeline and travel itinerary of the expedition cruise to the “Southern Lands”. The cruise started out from Ushuaia in Tierra del Fuego (Day 1) and visited South Georgia through to Day 7. Then the cruise turned back (Day 8) to again cross the Scotia Sea, but this time along the South Scotia Ridge. Destination the South Shetland Islands (Day 10) and the Antarctic Peninsula (Day 11), before finally returning to Ushuaia on Day 17.
- Antarctic on Le Lyrial (Days 1-7)
- Antarctic on Le Lyrial (Days 8-11)
- Antarctic on Le Lyrial (Days 12-17)
In February 2025 this particular itinerary no longer appeared on the Ponant website.
This blog posting tries to touch on a number of specific questions, namely:-
- What is the Scotia Sea, and Why Does it Exist?
- Why is the Antarctic Covered in Ice?
- Why does the Antarctic Remain Covered In Ice?
- Is Ice, Just Ice?
- Why is the Shetland Plate a Tectonic Battleground?
- What’s So Special About Sea Ice?
- How Do Whales Navigate in Ice-covered Regions?
- What Can Brown Bluff Tell Us About Volcanoes?
- How do Adélie and Gentoo Cohabit on Brown Bluff?
- How Many Penguins Did You Say?
- What is Krill?
- Can a Simple Stone Tell a Story?
And the visits to Penguin Island, Admiralty Bay, Brown Bluff, and Kinnes Cove.
There is a YouTube video summary of this particular trip.
The best travel summary I’ve ever seen is the 11-part Quest for the Antarctic Circle. The photos are superb.
I have numerous other blog pages covering other aspects of this exploration itinerary:-
- Planning a Cruise to the Antarctic
- My Antarctic Packing List
- Le Lyrial – A Luxury Expedition Cruise Ship
- Le Lyrial – Exploring Hidden Engineering
I have also reviews about my travel arrangements:-
- Air Dolomiti Embraer E195
- Lufthansa Business Class Lounge, Frankfurt
- Lufthansa Boeing 747-8 – Business Class
- Ministro Pistarini International Airport, Buenos Aires
- Hotel Meliã Recoleta, Buenos Aires
- Hotel Sofitel Recoleta, Buenos Aires
- Hotel Palladio, Buenos Aires
- Lufthansa Boeing 747-8 – First Class
- Lufthansa First Class Lounge, Frankfurt
And:-
I also have a separate blog post for my visit to the Museo Nacional de Bellas Artes, Buenos Aires.
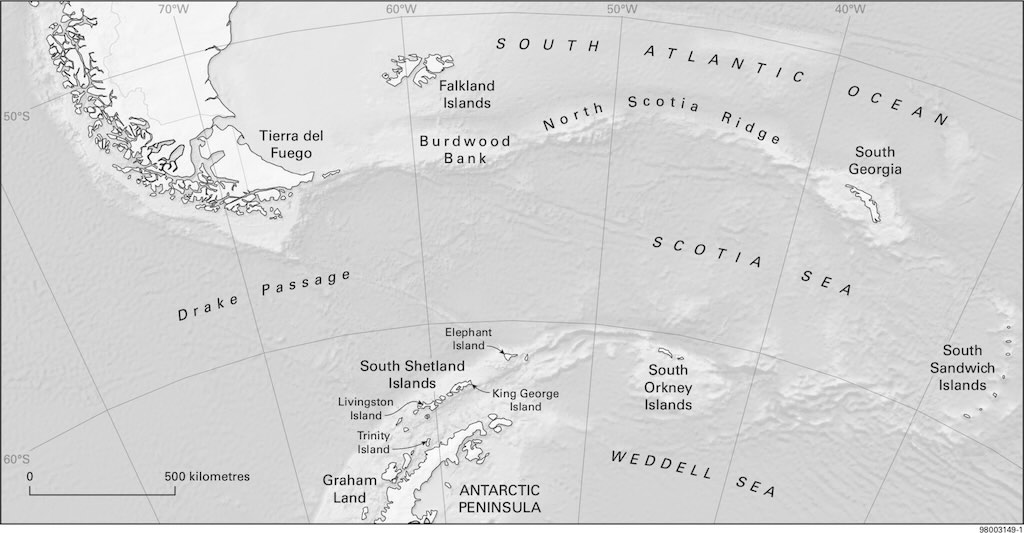
On December 2, 2024 we had visited Saint Andrews Bay and Gold Harbour in South Georgia (Day 7). The next part of the cruise (Days 8 and 9) involved leaving South Georgia and sailing towards the South Shetland Islands (Day 10), then on to Brown Bluff (Day 11) on the Tabarin Peninsula, the northeastern tip of the Antarctic Peninsula. Later we would have to cross the Drake Passage, back to Ushuaia (Days 15 to 17).
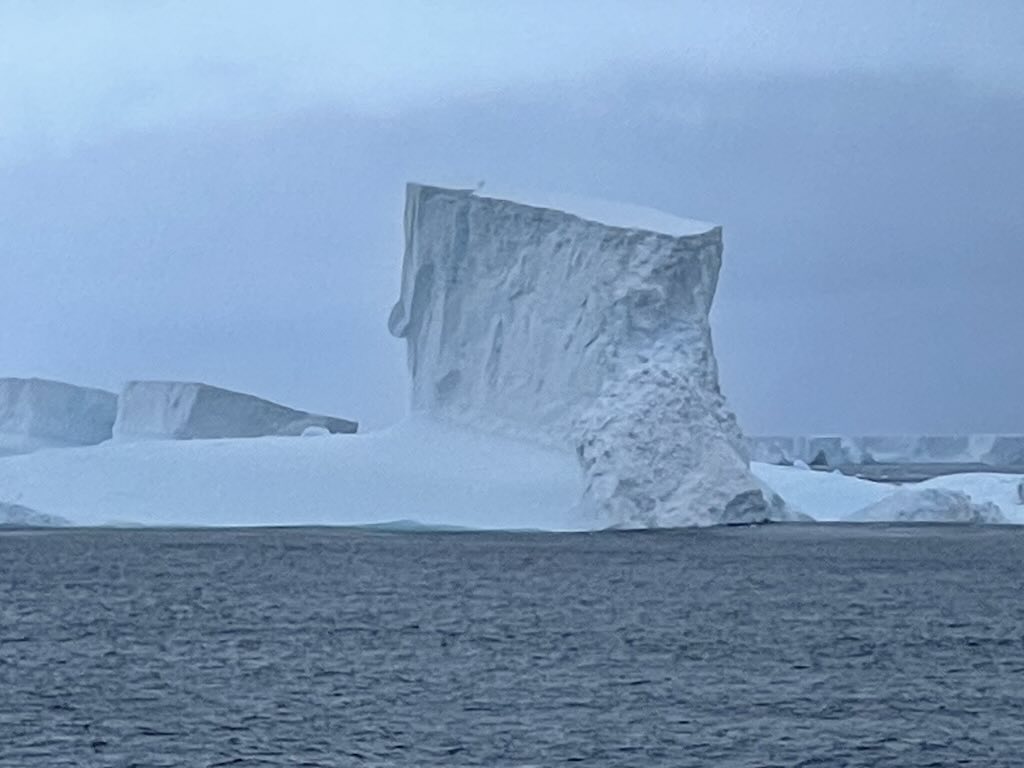
Travelling from South Georgia to the South Shetland Islands is a remarkable transition from the sub-Antarctic wildlife of Gold Harbour to the icy wilderness of the Antarctic Peninsula.
The ship returned to the Scotia Sea, which is one of the world’s most remote and biologically rich seas. After about a day of sailing, the ship entered the Antarctic Convergence, where colder Antarctic waters mix with warmer sub-Antarctic currents. Normally this is accompanied by a drop in water and air temperatures. This crossing is known for variable conditions, however we had wonderfully calm waters, although icebergs became more frequent as the ship approached the Antarctic Peninsula.
As the ship neared Brown Bluff, navigating through sea ice and ‘bergy bits‘ became crucial. With icebergs we start to also encounter a new world of terminology, e.g. bergy bits are medium to large fragments of ice.
The icebergs and floes (large packs of floating ice) made the passage slower but created an incredible landscape.
We also visited A23a, a large tabular iceberg which calved (breaking of ice chunks from the edge of a glacier) from the Filchner–Ronne Ice Shelf in 1986. Covering an area of about 3,900 sq km, it was called the largest iceberg in the world. We also had to make a significant detour around another iceberg-ice sheet.
The YouTube video “Drake Passage: The World’s Most Dangerous Sea Route” provides an accessible and interesting overview of the importance of the Drake Passage.
Calm night, woke around 04:00 local time.
Temperature -1°C and a 15km/h wind during the day.
Sunrise was at 02:57 and sunset at 21:25.
The day started with breakfast at 07:00, and I managed to avoid (again) the morning stretching at 08:00. I might add I also avoided a beginners lesson for line dancing at 11:30, and a wine tasting at 16:00, as well as another beginners lesson for the tango at 16:15.
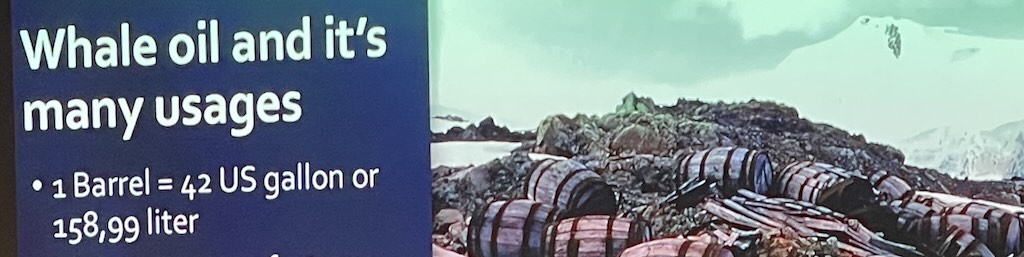
At 09:00 there was a talk “Modern Whaling History“, which was more like a modern look at past whaling practices.
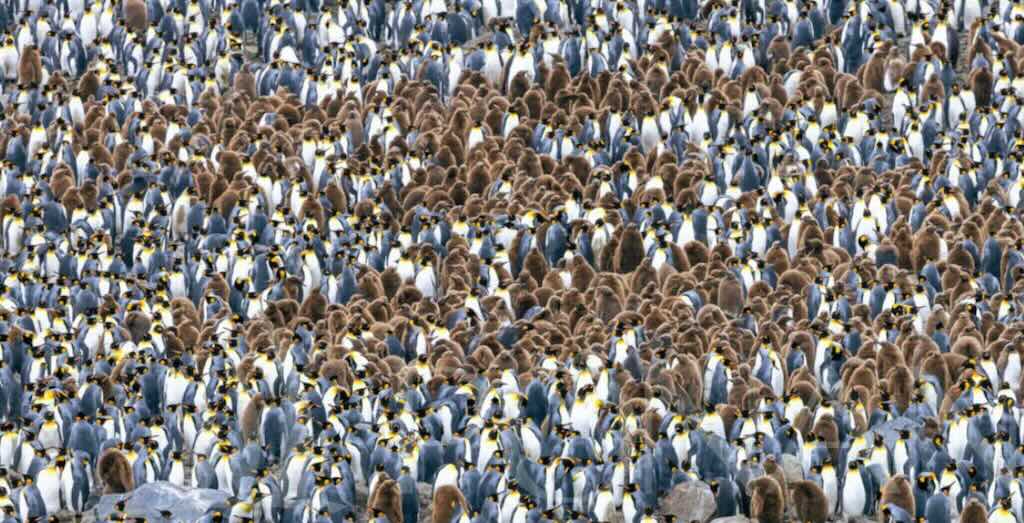
At 14:00 there was a talk “Super Birds: Penguins of the Peninsula“.
At 17:00 there was a General Knowledge Quiz. I participated in a somewhat larger group than last time, and again we were joint first with 14/20. We won the decider question which was “What is the colour of bear skin under the fur”. Our opponents answer was pink, and ours was correct with black. So we all won another Ponant keyring (which I treasured for a few weeks, before it disappeared).
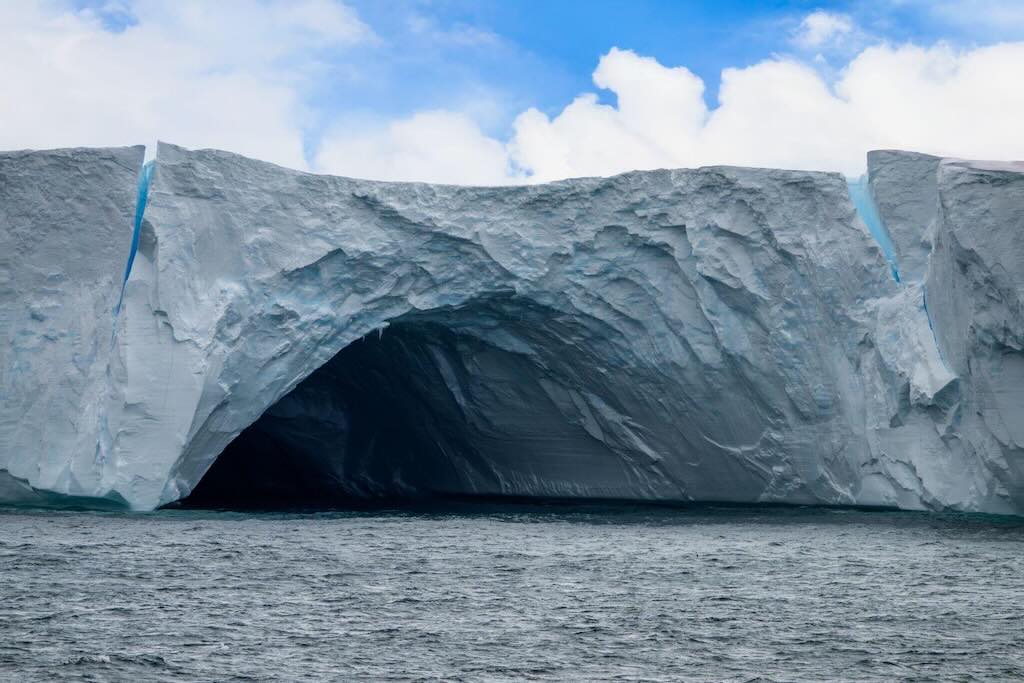
In the late afternoon-evening we sailed close to the largest iceberg in the world, the A23a.
As of February 2024, it had an area of approximately 3,900 square kilometres, which is about twice the size of London. With an estimated average thickness of 285 meters, its volume is about 1,113 cubic kilometres, and it has a mass of approximately 950 billion tonnes. Icebergs are composed entirely of freshwater ice, formed from compacted snow over millennia. Therefore, the mass of A23a consists solely of ice, without any liquid water content. As it drifts into warmer regions, it will gradually melt, releasing this freshwater into the ocean.
I guess all icebergs finally melt, but much depends on their trajectory. If it stays in cold water it could take several decades to melt.
Melting depends upon several factors. Warmer ocean temperatures accelerate melting, which depends on the size and surface area of an iceberg. Larger icebergs with more mass take longer to melt, but increased surface area can speed up melting due to more exposure to warmer water. Strong currents and waves can erode the iceberg faster, breaking it into smaller pieces that melt more quickly. Warmer atmospheric temperatures also contribute to surface melting. In warmer waters, the melting rate can reach 1-3 meters per day in thickness, so it might take anywhere from 2 to 10 years to completely melt.
At 18:00 there was a daily recap & briefing, about what had happened during the day, and what was planned for the next day.
I did not participate in the “70’s Party!” from 21:00-23:00. However I did take 50 mg dimenhydrinate just before bed.
What Is The Scotia Sea, and Why Does It Exist?
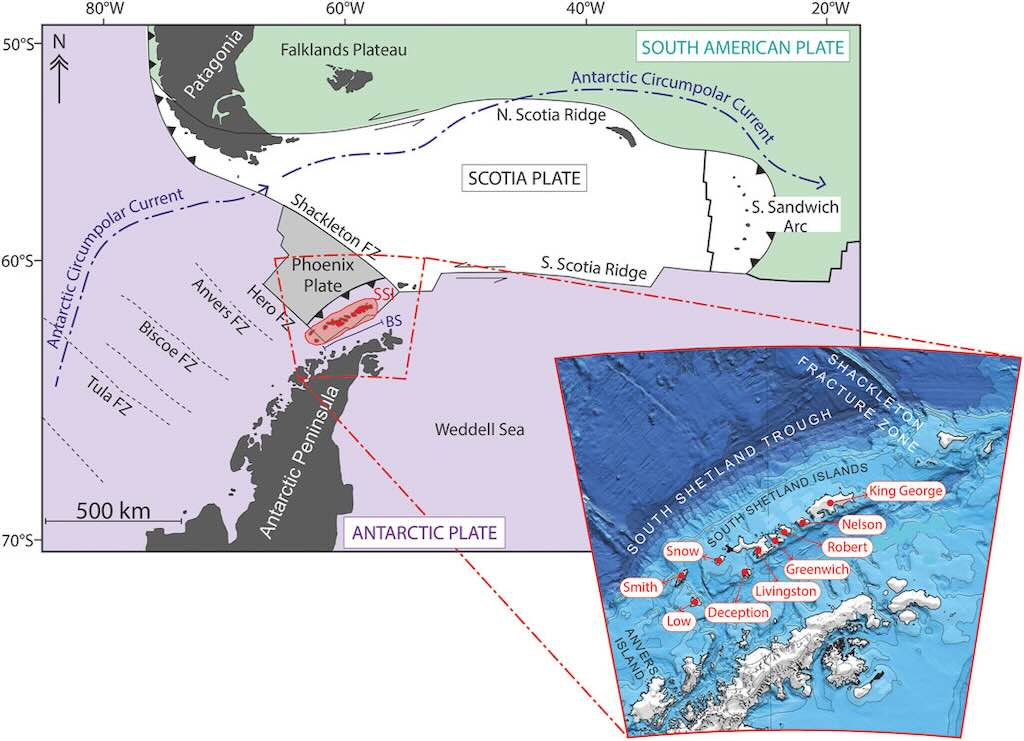
The above graphic shows some of the main features around the Scotia Sea and the Antarctic Peninsular. We sailed back from South Georgia toward the South Shetland Islands, where were would visit the islands of King George, Livingston and Deception, as well as the ‘main land’ of the Antarctic Peninsula.
The Scotia Sea, situated at the northern boundary of the Southern Ocean adjacent to the South Atlantic Ocean, is a region of intricate geological structures and dynamic oceanographic processes.
The Scotia Sea is predominantly underlain by the Scotia Plate, a minor tectonic plate bordered by the South American Plate to the north and the Antarctic Plate to the south. This plate’s formation is closely linked to the opening of the Drake Passage, which commenced in the early Eocene epoch (56 to 34 million years ago).
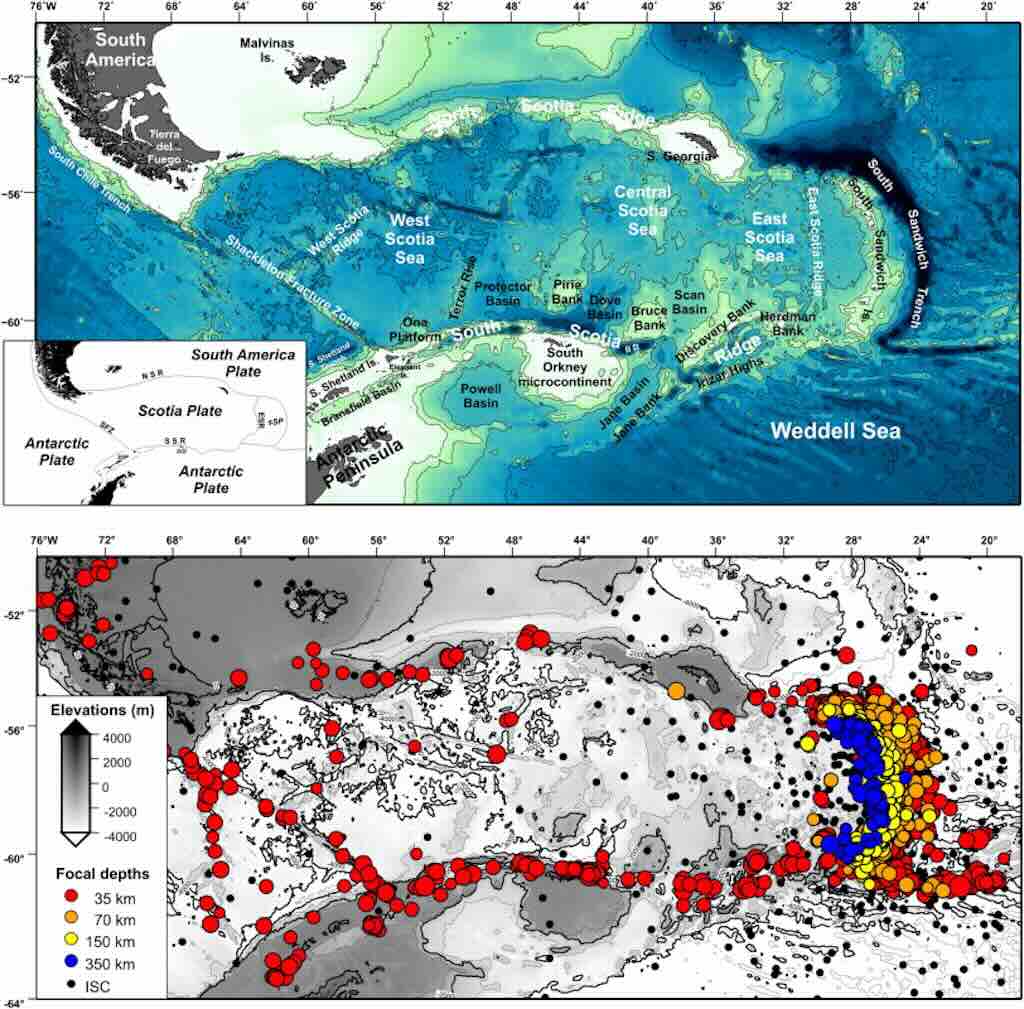
What are we looking at? In the top panel we have a physiographic map (landforms) of the Scotia Arc, with its main geological provinces. Bathymetric contours come from satellite-derived data presented in 1997. In the bottom panel we have the distribution of earthquakes in the Scotia Arc region. Epicentres and focal depths are collated from the Bulletin of the International Seismological Centre (ISC). Earthquakes in red are shallow, orange/yellow are intermediate and blue are deep. The smaller black circles represent earthquakes located by ISC.
The East Scotia Ridge is a mid-ocean ridge, where a new oceanic crust is being created as the Scotia Plate and the South Sandwich Plate move apart. This spreading process generates earthquakes along normal faults and transform faults in the ridge system.
The Scotia Sea is a highly active tectonic region, but the Pacific Ring of Fire, especially the Tonga-Fiji area, Japan, Sumatra, and Chile, surpasses it in terms of earthquake frequency, magnitude, and overall seismic energy release.
The tectonic activity in this region is characterised by complex interactions among several microplates, including the South Sandwich Plate. The northern boundary of the Scotia Plate, known as the North Scotia Ridge, is a transform fault zone exhibiting left-lateral (sinistral) motion relative to the South American Plate. This ridge encompasses various geological features such as the Burdwood, Davis, and Aurora Banks, as well as islands like South Georgia (our departure point).
A ‘transform fault’ is just where two tectonic plates slide past each other horizontally. These faults form at plate boundaries and cause earthquakes due to friction and stress buildup. A famous example is the San Andreas Fault in California. A fracture zone is a long, linear feature on the ocean floor that consists of inactive remnants of transform faults.
A ‘back-arc spreading centre’ (see below) is a region behind a volcanic arc where the Earth’s crust is pulled apart, creating new oceanic crust. This happens due to the movement of tectonic plates in a subduction zone (where one tectonic plate dives beneath another and sinks into the mantle). It often forms small seafloor ridges and basins. A trench is just a deep, narrow depression in the ocean floor, formed at a subduction zone where one tectonic plate sinks beneath another. Trenches are the deepest parts of the ocean and are associated with earthquakes and volcanic activity.
The southern boundary, termed the South Scotia Ridge, is another transform fault zone delineating the plate’s interaction with the Antarctic Plate. This ridge includes the South Orkney Islands and Elephant Island. The eastern margin of the Scotia Plate is defined by the East Scotia Ridge, an active back-arc spreading center resulting from the subduction of the South American Plate beneath the South Sandwich Plate along the South Sandwich Trench. This subduction process has given rise to the South Sandwich Island Arc, a chain of volcanic islands. The western boundary of the Scotia Plate is demarcated by the Shackleton Fracture Zone and the southern extension of the Chile Trench (the southern portion of the Peru–Chile Trench), where the Antarctic Plate is subducting beneath the South American Plate.
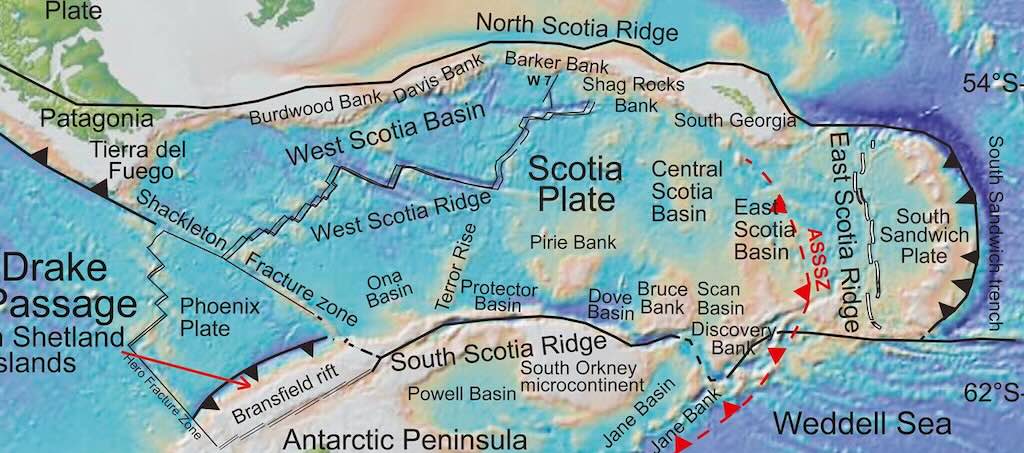
Within the confines of the Scotia Sea lie several basins, notably the West Scotia Basin and the Central Scotia Sea (see above graphic). The West Scotia Basin is an oceanic basin characterised by a seafloor predominantly covered with basaltic rocks, indicative of its volcanic origin.
The Central Scotia Sea, encompassing an area of approximately 120,000 square kilometers, exhibits varying depths ranging from 1,800 to 4,200 meters. The geological evolution of the Scotia Sea is marked by seafloor spreading events, particularly in the West Scotia Sea, which occurred between 30 and 6 million years ago. This tectonic activity has been instrumental in shaping the current configuration of the Scotia Plate and its surrounding features.
The Scotia Arc, an island arc system encompassing the North and South Scotia Ridges and the South Sandwich Islands, forms a discontinuous bridge between South America and Antarctica. This arc is a remnant of a once-continuous land connection between the two continents, which was disrupted by tectonic forces leading to the present-day configuration of the Scotia Sea.
The Scotia Sea plays a pivotal role in global ocean circulation, primarily due to the presence of the Antarctic Circumpolar Current (ACC). The ACC is the world’s most voluminous ocean current, facilitating the exchange of water masses between the Atlantic, Pacific, and Indian Oceans. Within the Scotia Sea, the ACC is characterized by several frontal systems, including the Sub-Antarctic Front, the Polar Front, and the Southern ACC Front. These fronts are associated with sharp gradients in temperature and salinity, influencing the distribution of marine species and biogeochemical processes.
The interaction of the ACC with the complex bathymetry of the Scotia Sea, including its ridges and basins, leads to the formation of mesoscale eddies and localised upwelling zones. These dynamic processes enhance nutrient mixing, supporting a rich and diverse marine ecosystem. Deep-water currents in the Scotia Sea are influenced by the inflow of Weddell Sea Deep Water (WSDW) and Circumpolar Deep Water (CDW). The mixing of these water masses contributes to the ventilation of the deep ocean and plays a role in the global thermohaline circulation. The complex interplay of currents and the region’s topography also affects the drift patterns of icebergs calving from Antarctic ice shelves, posing navigational challenges.
Navigating the Scotia Sea can be challenging because of the extreme environmental conditions. The region is notorious for its severe weather patterns, including frequent storms and high winds, which can lead to rough seas. The confluence of cold Antarctic waters with relatively warmer sub-Antarctic waters can result in dense fog, reducing visibility. The presence of icebergs and sea ice, especially during the austral winter and spring, adds to the navigational hazards.
Mariners must be equipped with accurate and up-to-date nautical charts that detail the sea’s bathymetry, including its numerous submerged features such as seamounts and ridges. Real-time monitoring of oceanographic conditions, including current patterns and weather forecasts, is essential for safe passage. Technological tools, such as the Automatic Identification System (AIS), aid in tracking vessel movements, enhancing situational awareness, and preventing collisions. Platforms like ShipTraffic.net provide live maps displaying the current positions of ships, along with shipping routes and traffic density in the region. Given the ecological significance of the Scotia Sea, there are international regulations and guidelines in place to minimize the environmental impact of shipping activities. These include measures to prevent oil spills, reduce emissions, and avoid disturbances to marine wildlife.
4 December 2024 - Sailing Towards the Antarctic Peninsula (Day 9)
Calm night, woke around 05:00 local time.
Temperature -1°C and a 20km/h wind during the day.
Sunrise was at 02:54 and sunset at 22:54.
The day started with breakfast at 07:00, and (as usual) I managed to avoid the morning stretching, the afternoon beginners lesson for cumbia dancing, and the 21:30 show “Around the World”.
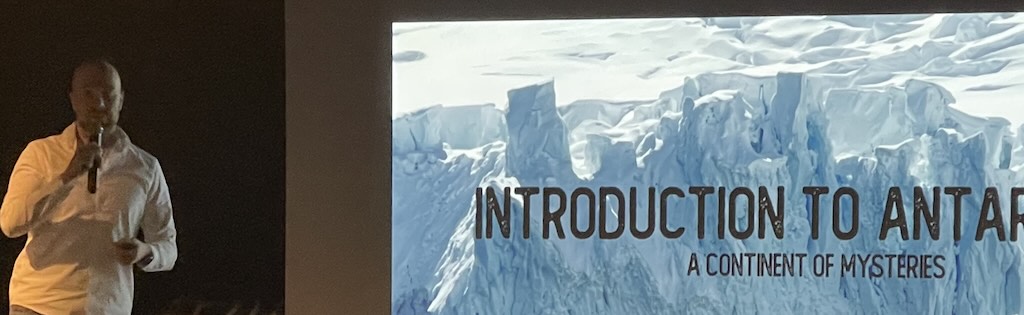
At 10:15 there was a talk on “The White Continent“.
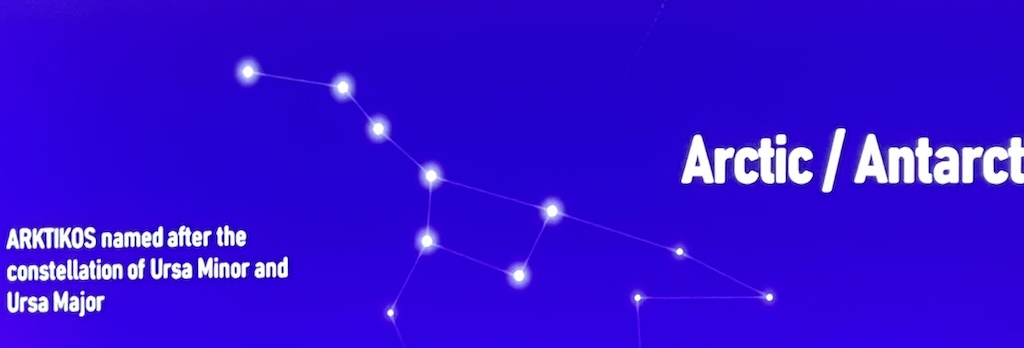
At 14:00 there was a talk “Antarctica: Frozen in Time“, subtitled “Glaciology Concepts and Theories”.
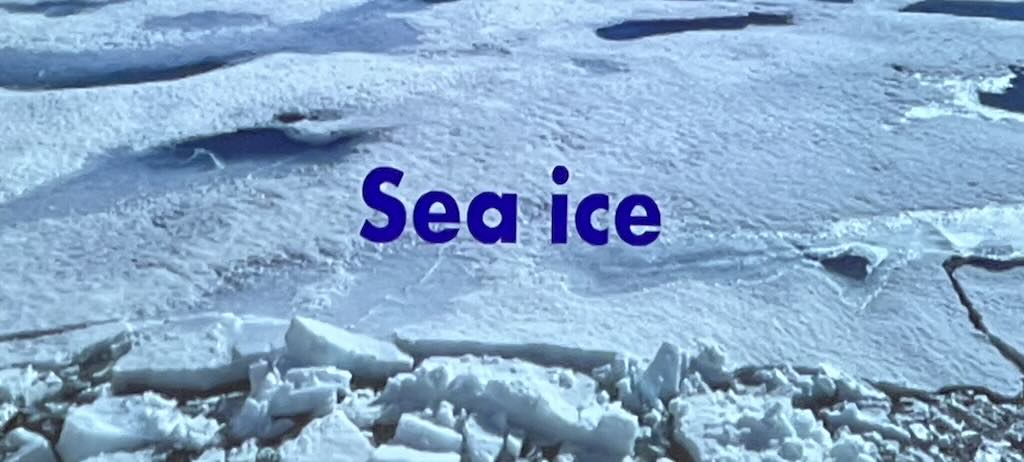
At 17:45 there was a daily recap & briefing, about what had happened during the day, and what was planned for the next day.
I took 50 mg dimenhydrinate just before bed.
Why is the Antarctic Covered In Ice?
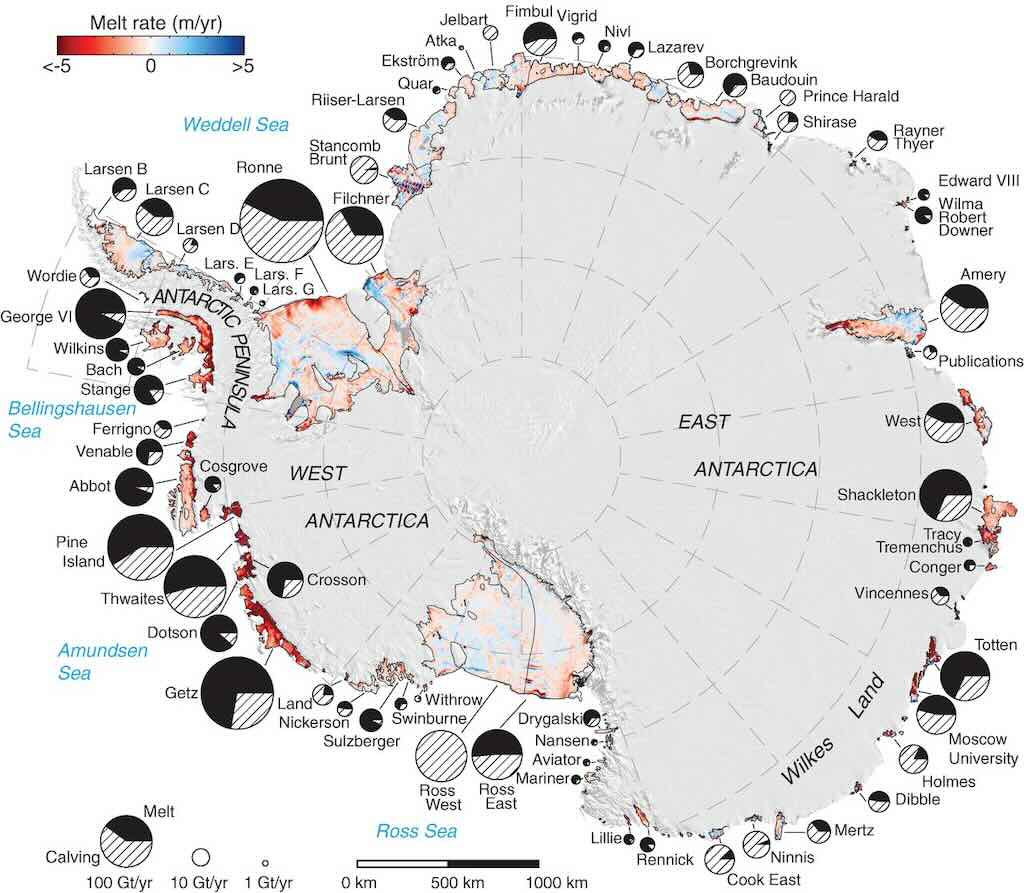
Before we look at the past, let’s take a quick look at the present.
The ice shelves and floating ice tongues that surround Antarctica cover more than 1.5 million square kilometres, approximately the size of the entire Greenland Ice Sheet. Conventional wisdom has held that ice shelves around Antarctica lose mass mostly by iceberg calving, but recently it has become increasingly clear that melting by a warming ocean may also be important.
In fact, basal melt of 1325±235 gigaton per year (Gt/year) now exceeds a calving flux of 1089±139 Gt/year, now making ice-shelf melting the largest ablation process in Antarctica.
Let’s now step back in time…
Between 500–250 million years ago (Paleozoic Era) the Antarctica was part of the supercontinent Gondwana, and was covered in forests and inhabited by early life forms. At the time Gondwana included South America, Africa, Antarctica, Australia, Zealandia, Arabia, and the Indian subcontinent, in one massive landmass.
About 180 million years ago (Jurassic Period) Gondwana began breaking apart, caused by hot mantle plumes (rising columns of molten rock) building up beneath it. These plumes created upward pressure, causing the lithosphere (Earth’s rigid outer shell) to stretch and crack. But the Antarctic was still connected to South America and Australia.
Between 100–66 million years ago (Cretaceous Period) Antarctica had a warm, forested climate with dinosaurs, rivers, and a temperate ecosystem. For example, large coal deposits found in Antarctica show that it once had dense forests. These coal beds date back to the Carboniferous (~300 million years ago) and later periods.
About 34 million years ago (Eocene-Oligocene Transition) Antarctica, fully separated from South America and Australia, had finally drifted into its current isolated position over the South Pole, allowing the Antarctic Circumpolar Current (ACC) to form, isolating it from warm waters. The separation was caused by plate tectonics, specifically mantle convection, rifting, and seafloor spreading. This process took tens of millions of years and was driven by forces deep within the Earth’s mantle.
Mantle Convection means slow-moving convection currents that push and pull tectonic plates apart (and continue to do so, even today). Rifting just means the cracks and breaks that occur in the Earth’s Crust, and around 55 million years ago, they would have been the first signs of the continent breaking up. Long cracks (rift zone) developed and magma would have rose up from the mantle, forcing the continents apart. As the rifts widened, a new oceanic crust formed between Antarctica and Australia, creating the Southern Ocean. The Tasman Gateway (between Antarctica and Australia) fully opened around 34 million years ago, allowing ocean water to flow between them. And a similar process occurred between Antarctica and South America, leading to the opening of the Drake Passage around the same time. So the Antarctic Circumpolar Current (ACC) was created, cutting Antarctica off from the warmer ocean currents. From then on ice sheets expanded rapidly, covering Antarctica and making it the frozen continent it is today. This dramatic cooling event was driven by several key factors:-
Continental drift & isolation of Antarctica
- Separation from South America & Australia: Around 34 million years ago, Antarctica drifted away from South America and Australia. The shift in deep-sea sediment composition and fossil evidence supports the formation of the Antarctic Circumpolar Current (ACC). And sediment cores from the Southern Ocean show changes in ocean circulation consistent with the opening of these seaways.
- Formation of the Antarctic Circumpolar Current (ACC): The opening of these ocean pathways allowed a powerful current to form around Antarctica, isolating it from warmer tropical waters and trapping cold waters around the continent. Firstly, ocean sediment cores from the Southern Ocean and Antarctica contain an abrupt increase in ice-rafted debris at ~34 million years ago, indicating that glaciers and ice sheets were growing and calving into the ocean. Also there was a sharp increase in ‘delta-O-18’ levels (the ratio of the heavy oxygen isotope O-18 to the light oxygen isotope O-16 in a sample, compared to a standard) in Foraminifera (a microscopic marine plankton) which store oxygen isotopes in their shells. This suggests a major cooling event, probably due to the growth of large ice sheets, since ice preferentially removes the lighter oxygen isotope (O-16) from the ocean. There was also a simultaneous shift in ‘delta-C-13’ the ratio of the heavy carbon isotope C-13 to the light carbon isotope C-12 in a sample compared to a standard. This suggests changes in the carbon cycle, possibly due to a reduction in carbon-dioxide levels.
- Before the freeze: Antarctica had forests with warm-climate plants, such as beech trees and ferns. Fossil pollen and plant remains show a rapid transition from forests to tundra vegetation around 34 million years ago. After this point, cold-adapted plants became dominant, and eventually, most plant life disappeared under ice. Oxygen estimates from ancient soil carbonates and marine algae suggest a significant drop in atmospheric carbon-dioxide levels from ~1,000 ppm to ~600–750 ppm just before the Antarctic glaciation. And computer models of climate sensitivity indicate that below ~600 ppm carbon-dioxide, global cooling occurs and ice sheets can form. The reason for this decline is still debated, but it may be linked to reduced volcanic carbon-dioxide emissions and increased weathering of rocks, which pulls carbon-dioxide from the atmosphere.
Growth of ice sheets
- As Antarctica cooled due to isolation and lower carbon-dioxide, ice sheets started forming and expanded rapidly within a few hundred thousand years.
- Once some ice started forming, it reflected more sunlight (high albedo), cooling the region further.
- Ice cores and sediment records suggest that by 33.5 million years ago, Antarctica was permanently glaciated.
Global climate shift
- This event marked the end of the warm, tropical climate that had dominated the planet and the beginning of the modern icehouse climate we are still in today.
- Other regions of the world also experienced cooling, leading to the spread of grasslands and changes in ocean circulation.
Subsequent research has provided further insights into this debate. A 2024 study reinforced the notion that the ACC’s formation postdated the onset of Antarctic glaciation. This study emphasised that the oceanographic current developed after the glaciation had already begun, challenging the idea that the ACC was a driving factor in the initial ice sheet formation.
Additionally, a 2017 study from McGill University proposed a synthesis of competing theories regarding the origin of Antarctic glaciation. This research highlighted the complexity of climate change and suggested that multiple factors, including declining atmospheric carbon-dioxide levels and tectonic shifts, likely contributed to the rapid formation of the Antarctic ice sheet around 34 million years ago.
Why Does the Antarctic Remain Covered In Ice?
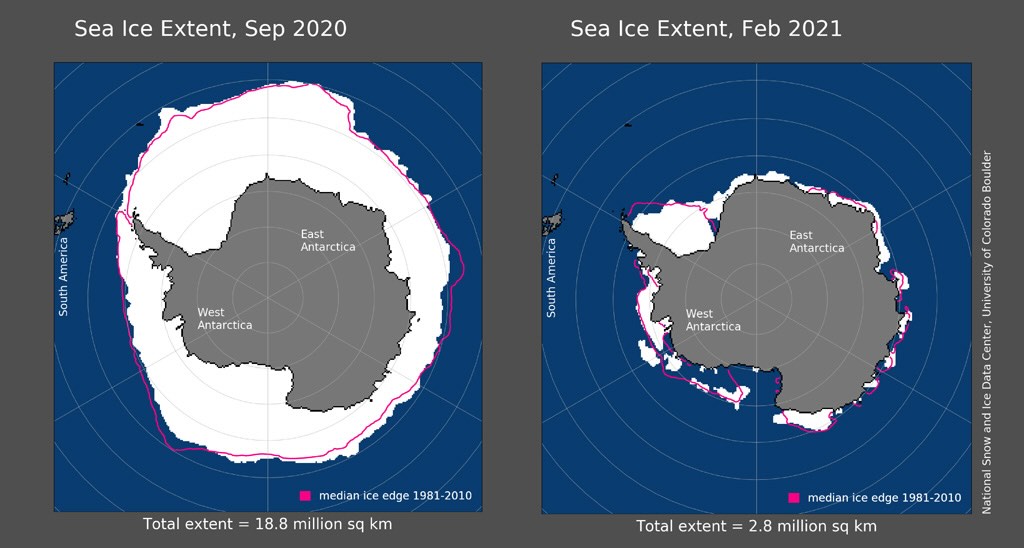
The sharp decline in Arctic sea ice over recent decades has become one of the most enduring images of the Earth’s warming climate. Yet, at the same time, the sea ice changes at the Earth’s south pole have been much less clear-cut. The annual fluctuation in Antarctic sea ice is one of the largest seasonal changes in surface cover on the planet. It has huge knock-on effects for the global climate and marine ecosystems.
In winter, Antarctica effectively doubles in size as more than 15 million square kilometres of the ocean freezes. While the Arctic is hemmed in by continents that limit seasonal sea ice growth, the Antarctic is surrounded by kilometre-after-kilomtre of water where sea ice can form.
However, because sea ice in Antarctica spreads to lower, warmer latitudes, less will then survive in the summer as temperatures rise. On average, only around 15% of Antarctic sea ice lasts from one year to the next, unlike around 40% for the Arctic.
The above maps show the extent of Antarctic sea ice at the 2020 winter maximum (left) and the 2021 summer minimum (right).
Even though Earth has experienced warm periods since Antarctica froze, the ice has remained because:-
Extreme cold & isolation
- Antarctica is the coldest place on Earth, with temperatures reaching -80°C in winter.
- The Antarctic Circumpolar Current (ACC) still prevents warm air and water from reaching the continent.
- The high elevation (Antarctica has an average elevation of 2,500m) also contributes to its cold climate.
Ice sheet thickness & stability
- Antarctica’s ice sheet is up to 4.8 km thick, which makes it very stable.
- Even during warm periods (e.g., the Pliocene, ~3 million years ago), only parts of the ice sheet melted.
- The East Antarctic Ice Sheet (EAIS) is especially stable and has persisted for millions of years.
Weak sunlight at the poles
- Because of Earth’s tilt, Antarctica receives little to no sunlight in winter.
- Even in summer, the Sun is low in the sky, meaning less solar energy reaches the surface.
- This prevents large-scale melting, unlike in the Arctic, where sea ice is more vulnerable.
Is ice, Just Ice?
The obvious answer in no, there are many types of ice.
It not difficult to come up with 10-20 different types of ice, ranging from snow and hail, through glaciers and permafrost, to the impressive iceberg and the fantastic colour of blue ice.
And everyone knows that the Inuit-Yupik (Eskimo-Aleut languages) may collectively have hundreds of words for different forms of ice, ranging from Sikuliaq for newly formed ice, to Sikutuq for very thick, old ice.
Is all ice from freshwater? Meaning water containing low concentrations of dissolved salts. Here the answer is mostly yes, but a bit, no!
Most natural ice on land is freshwater, because when water freezes, it excludes salts and impurities. This includes:
- Glaciers & Ice Sheets – The Antarctic Ice Sheet and Greenland Ice Sheet, as well as glaciers worldwide, are almost entirely fresh water.
- Icebergs – Since they calve (break off) from glaciers or ice shelves, they are made of fresh water.
- Lake Ice & River Ice – Freshwater lakes and rivers freeze in cold climates, forming freshwater ice.
However ice can form from ocean water, which is salty. Nevertheless when seawater freezes, most of the salt is left behind, but some traces remain:
- Sea Ice – Forms from frozen ocean water and initially contains some salt, though much of it drains out over time.
- Young Sea Ice – More salty, as brine (saltwater pockets) is still trapped in the ice.
- Multi-Year Sea Ice – Becomes almost fresh water over time as the salt drains out.
- Brine Pockets – In sea ice, small pockets of concentrated salty water remain trapped for a while before they eventually seep out.
And there is the special case of Anchor Ice that forms on the seafloor in very cold ocean water, and which can contain some salt.
We often think of the Antarctica (the continent) as just being covered in ice. Which is true, but which is also a massive over-simplification.
It’s true because the land is covered in the Antarctic Ice Sheet. What people forget is that the total surface area is ~14 million square-kilometres, so roughly the size of the US and Mexico combined. The average thickness is just over 2 kilometres, but can be nearer 5 kilometres in places. That’s a volume of ~26.5 million cubic-kilometres, which represents about 60–70% of the world’s fresh water.
That’s ~26.5 quadrillion metric tons, and that why it pushes down the Antarctic continent. In some places the land is depressed by hundreds of meters below sea level. This effect is called an isostatic depression, and if the ice melts, the land would slowly rises back up in a process called glacial isostatic adjustment (GIA).
If the Antarctic ice sheet melted completely, it would cause global sea levels to rise by ~58 meters. And in addition, the land beneath the melted ice would eventually rise by hundreds of meters, but this process would take thousands of years.
On top of that the gravitational pull of Antarctica’s ice affects global ocean currents by slightly pulling seawater toward it. But if the ice sheet melted, the gravitational pull would weaken, and the water would be redistributes globally. Paradoxically, sea levels around Antarctica would actually drop, but rise dramatically elsewhere, particularly in the Northern Hemisphere (due to gravitational shifts). The movement of such a large mass of water into the ocean would cause a slight shift in Earth’s rotation and shift Earth’s geographic pole, and could slightly alter the length of a day (on the scale of microseconds). We won’t mention the changes to ocean salinity, currents and jet streams, and the destabilisation of numerous ecosystems around the world.
And we should not forget that some of the ice is over 1 million years old. In fact the ancient air bubbles help scientists study past climate conditions.
Reading the above suggests that if the Antarctic ice sheet melted, then sea levels would change around the world. But by how much?
The complete melting of the Antarctic Ice Sheet is not expected in the immediate future. However, certain regions, particularly the West Antarctic Ice Sheet (WAIS), are showing signs of increased vulnerability. Some models predict that several basins within the WAIS could undergo complete collapse before the year 2200. WAIS holds 2.2 million cubic-kilometres of ice, or the equivalent to a rise of ~3.3 meters in sea levels. But factoring in both the melted water and the change in gravity, the result would probably be a drop of about 1 meters in the Antarctic and a rise in sea levels in excess of 3 meters in London and New York. As a final thought on the topic, today Antarctica is losing ~150 billion tons of ice per year. If WAIS were to totally disappear by 2200, it would have to lose around 70-80 time more ice per year than the above figure.
The South Shetland Islands
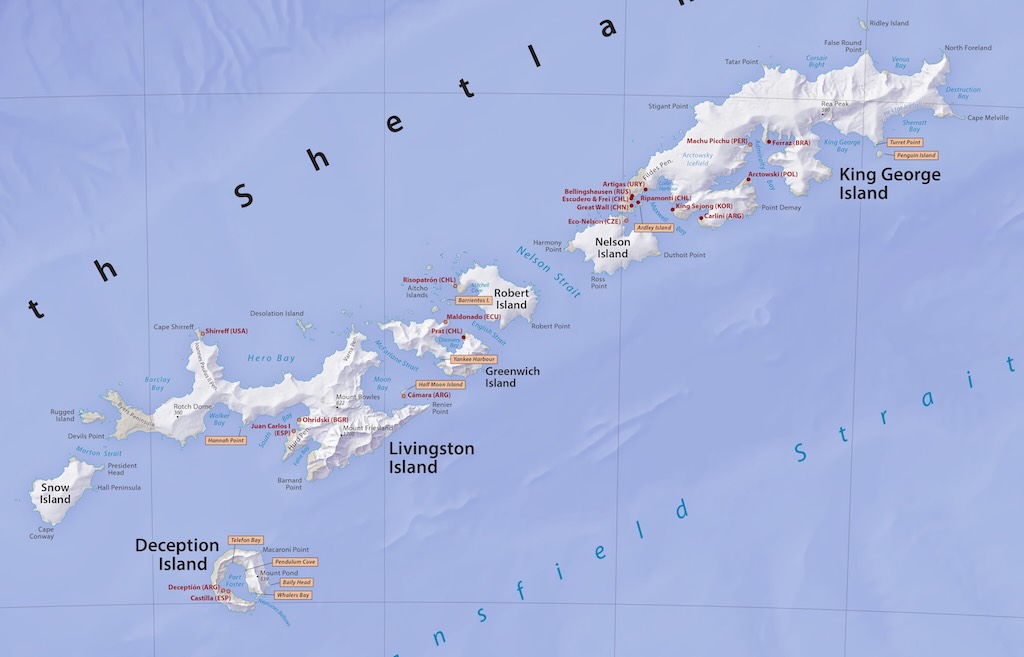
Leaving South Georgia our destination was the South Shetland Islands and the Antarctic Peninsula.
The British Antarctic Territory published in 2019 an article entitled “Two hundred years since the discovery of the South Shetland Islands“, highlighting the first sighting and first landing in 1819.
The South Shetland Islands are interesting because they combine geographical, ecological, and geopolitical characteristics. First they sit between the Antarctic and sub-Antarctic climatic zones, and are influenced by both polar and temperate systems. The islands sit on an oceanic boundary, where cold Antarctic waters meet warmer sub-Antarctic waters, fostering nutrient upwelling, which supports an unusually high degree of marine biodiversity. The islands are rich breeding grounds for seabirds (e.g. penguins, petrels) and seals (e.g., fur seals, elephant seals). The nutrient-rich waters are ideal for studying food web dynamics, krill populations, and predator-prey interactions in polar marine ecosystems.
The islands are highly sensitive to climate change, with rapidly warming temperatures, retreating glaciers, and shifting species distributions. Monitoring these changes provides critical data for global climate models.
The islands are also part of an active tectonic region, sitting along the South Shetland Trench where the Antarctic Plate meets the South American Plate. The islands host volcanic activity (e.g., Deception Island, a caldera with active geothermal systems) and glaciated landscapes, making them a natural laboratory for studying Earth’s geological processes. Ice cores and sediments from the region serve as archives of historical climate data, helping reconstruct past climate variability and predict future changes.
The islands are managed as part of the Antarctic Specially Protected Areas (ASPAs), and host numerous research stations operated by countries such as Argentina, Chile, China, and Russia.
The Shetland Plate looks to be a small, relatively obscure tectonic plate (a tectonic microplate) located near the Scotia Plate in the South Atlantic, just off the Antarctic Peninsula. What makes it particularly interesting isn’t just its existence but rather its dynamic interactions with surrounding plates, its geological history, and its role in shaping the surrounding region.
The Shetland Plate is a microplate, meaning it was once part of a larger tectonic structure before breaking off due to complex plate movements. It is sandwiched between the Antarctic Plate and the Scotia Plate, and its movements are heavily influenced by the surrounding tectonic forces. In fact the islands are an above-sea-level expression of these tectonic forces, with active rift zones and volcanic activity. The Shetlands Islands also played a key role in the opening of the Drake Passage, which separated South America from Antarctica around 30-50 million years ago.
The plate boundary is highly active, producing earthquakes and volcanic activity (e.g. we visited Deception Island, which is an active volcano in the South Shetlands).
Why is the Shetland Plate a Tectonic Battleground?
The Shetland Plate is a seismically active microplate due to its complex interactions with surrounding plates, especially in the Bransfield Strait and the South Shetland Trench. Earthquakes in this region are caused by subduction (where a heavier plate dives beneath another), back-arc spreading (when the overriding plate is stretched, causing seafloor spreading), and transform faulting (a fault along a plate boundary where the motion is predominantly horizontal), making it a tectonic hot spot despite its small size.
The seismicity of the Shetland Plate is concentrated in three key areas:-
The South Shetland Trench (subduction zone)
- The Antarctic Plate is subducting beneath the Shetland Plate at the South Shetland Trench. Subduction occurs when one tectonic plate sinks beneath another into the mantle. This process generates earthquakes as the descending plate scrapes against the overriding plate, builds up stress, and then suddenly releases energy along the subduction zone.
- This process generates moderate to strong earthquakes (typically M5–M7), but it’s considered a slow subduction zone, meaning it’s not producing megathrust earthquakes (M9 or more) like those in the Pacific’s Ring of Fire.
- There is debate among geologists about how active the subduction is today. Some believe it’s transitioning into more of a slip-strike system, which would alter the seismic profile over time (i.e. potentially less intense earthquakes compared to subduction zones).
The Bransfield Strait (back-arc spreading)
- This is one of the most interesting seismic zones near the Shetland Plate.
- The Bransfield Strait is a back-arc basin, meaning it’s stretching as a result of plate motion.
- There is active rifting here, meaning the seafloor is spreading apart, leading to shallow earthquakes and occasional volcanic activity (e.g. Deception Island).
- Many earthquakes here are low to moderate magnitude (M4–M6) but are frequent, indicating continuous tectonic stress.
The Transform Faults (Scotia Plate interaction)
- The Shetland Plate is squeezed between the Scotia Plate and the Antarctic Plate, creating strike-slip faults (plates slide past each other horizontally).
- These faults, similar to the San Andreas Fault, generate horizontal movement, producing frequent but smaller earthquakes (M3–M5).
Earthquakes are classified by magnitude (M) using the Moment Magnitude Scale (Mw):
- M1.0 – M2.9: Micro – Generally not felt.
- M3.0 – M3.9: Minor – Often felt, but rarely causes damage.
- M4.0 – M4.9: Light – Noticeable shaking, minimal damage.
- M5.0 – M5.9: Moderate – Can cause damage to weak structures.
- M6.0 – M6.9: Strong – May cause significant damage in populated areas.
- M7.0 – M7.9: Major – Widespread damage, potential loss of life.
- M8.0+: Great – Severe destruction over large areas, massive impact.
There is no theoretical upper limit, but the largest recorded quake was M9.5 (1960, Chile).
For comparisons sake, M2 is about the same a 1kg TNT explosion, whereas M6 is about the same as 1,000 tons of TNT, and M8 about the same as 1 million tons of TNT. The M9.5 Chile earthquake (1960) released energy equivalent to 178 gigatons of TNT. The Hiroshima “Little Boy” was ~15 kilotons of TNT, the Nagasaki “Fat Man” was ~21 kilotons of TNT, the largest ever tested, the Russian “Tsar Bomba“, had a yield of ~50 megatons of TNT.
The region has seen multiple M5+ earthquakes in recent decades, with some seismic swarms occurring in the Bransfield Strait. Seismic swarms near Deception Island (where we visited) are often linked to magma movement beneath the crust, meaning some of these quakes could be volcanic in origin rather than purely tectonic.
In late 2020 and early 2021, the Bransfield Strait experienced an intense earthquake swarm, with over 85,000 events recorded near the Orca Seamount, a submarine volcano. The largest of these events reached M6.9. This swarm is believed to be associated with magmatic intrusions and rifting processes in the region.
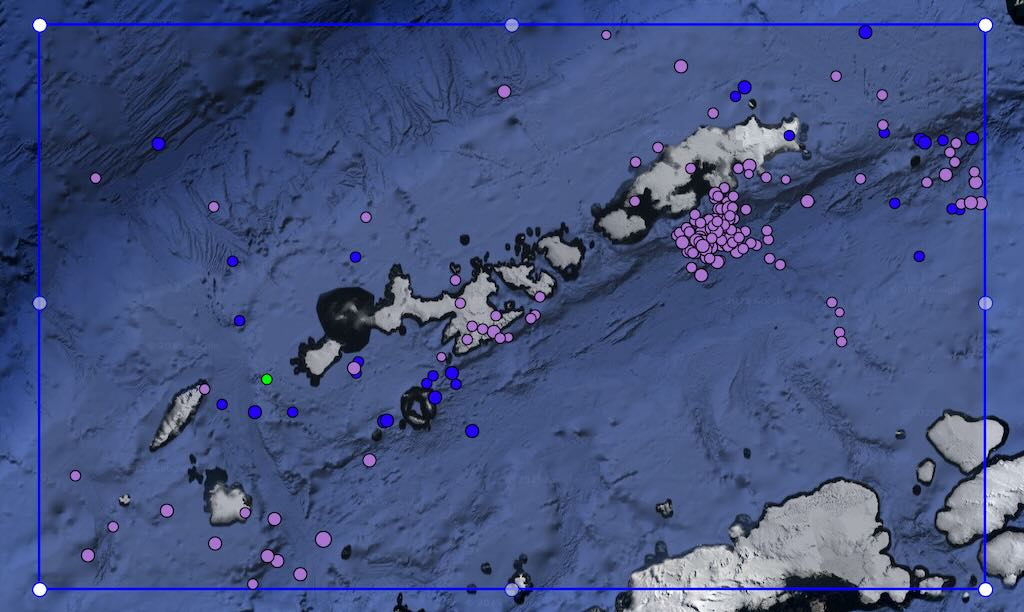
There is a freely available, interactive earthquake map of the South Shetland Islands region through the IRIS (Incorporated Research Institutions for Seismology) Interactive Earthquake Browser. This tool allows you to explore seismic events in the area, providing detailed information on each recorded earthquake.
The above graphic is of the most recent 200 seismic events, defined within the blue frame. The size of the dot indicates the force of the event (usually between M3 and M4), and the colour purple is to a depth of 33 km, and blue to a depth of 70 km. The one green dot was nearer the surface.
Calm night, woke around 04:30 local time.
Temperature -2°C and a 7-12km/h wind during the day.
Sunrise was at 03:07 and sunset at 22:19.
The day started with breakfast at 07:00, and I again managed to avoid the morning stretching, the afternoon beginners lesson in Bollywood dancing, and the “White Evening” that followed the “Officer’s Dinner!”.
King George Island
It’s probable that King George Island was first sighted in February 1819 by British explorer William Smith, during his discovery of the South Shetland Islands. Fabian Gottlieb von Bellingshausen independently confirming its existence in 1820. It was William Smith that named it “King George Island”, according to the tradition of explorers to name new locations after reigning monarchs or patrons.
King George Island is the largest of the South Shetland Islands, covering approximately 1,150 square-kilometres. The island has a polar climate with ice-covered terrain and is surrounded by glaciers. But this is not the full story!
The island is an important sanctuary for wildlife, and this is why it’s home to a dozen research stations from multiple countries, making it a central base for Antarctic scientific collaboration.
This begs the question, why does the island support a diverse range of wildlife despite its harsh environment. It is a breading ground for Adélie, Gentoo, and Chinstrap penguins, with large colonies concentrated near ice-free areas. Antarctic petrels, Wilson’s storm petrels, and south polar skuas are common, and the imperial shag, a type of cormorant, nests along the rocky shores. Weddell Seals are often found on the sea ice around the island, as are leopard seals (who hunt penguins), and Crabeater and Elephant Seals frequently use the island’s beaches for breeding and resting. Humpback, minke, and orca are often seen in the surrounding waters, particularly during the summer feeding season. The island also has mosses and lichens that grow in ice-free areas, and are essential for soil development and provide a habitat for invertebrates.
And it all starts with the fact that the Southern Ocean surrounding King George Island is rich in nutrients, thanks to upwelling currents. These currents bring nutrient-dense waters from the ocean’s depths to the surface, fuelling the growth of phytoplankton, the primary food source for krill. And Antarctic krill form the basis of the food web. They support the island’s seabirds, seals, and whales. The island’s proximity to ice-free areas and seasonal sea ice provides an optimal environment for phytoplankton blooms. During the summer, long daylight hours and melting ice release trapped nutrients, creating a boom in phytoplankton growth, which krill feed on.
King George Island lies near the Antarctic Circumpolar Current (ACC), the world’s strongest ocean current. This current and localised eddies help concentrate krill and their food sources in the waters around the island. Krill rely on sea ice for breeding and feeding. Juvenile krill often feed on algae that grow on the underside of the ice. The seasonal cycle of sea ice around King George Island provides a critical habitat for these activities.
So the island has large colonies of seals, penguins, and whales because they are attracted to places that have an abundant krill population.
Another reason why the island hosts numerous research stations is that it’s one of the most accessible Antarctic islands. There are relatively extensive flat areas suitable for infrastructure development, including scientific facilities and logistical support. For example, it has an airstrip (Teniente R. Marsh Airport) near the Chilean research station, providing a critical entry point for researchers and supplies. On top of all that the breeding and feeding sites are also relatively accessible, making it a focal point for conservation efforts.
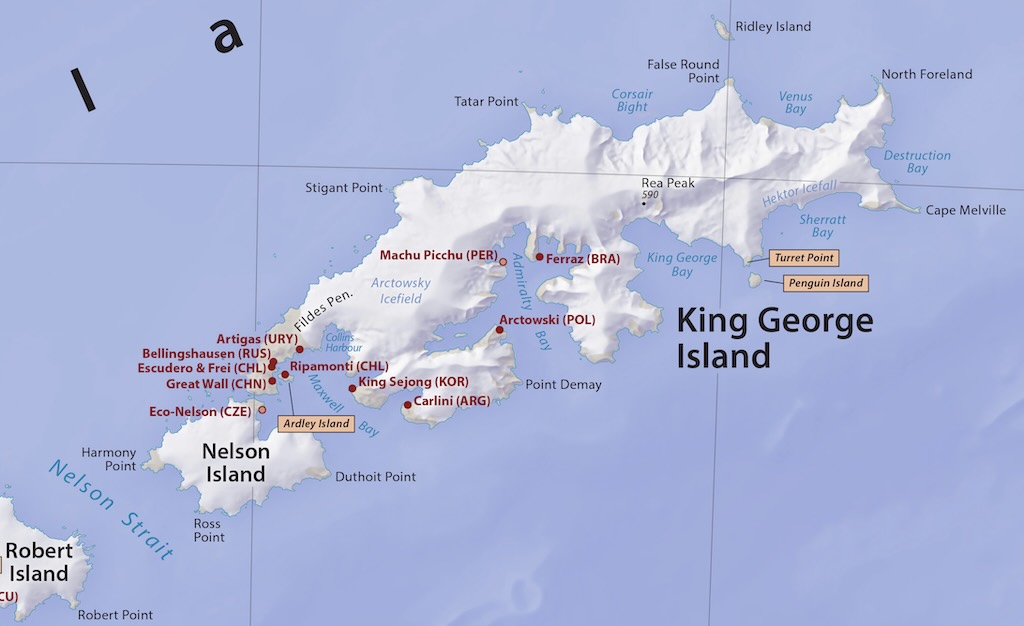
The closed red dots are permanent, year-round research stations, and the open red dot is a permanent, summer-only research station. The three orange boxes are popular tourist landing sites.
The first permanent base on King George Island was established by Chile in 1947, with the construction of Base Presidente Gabriel González Videla (now inactive). This was part of Chile’s efforts to assert territorial claims in Antarctica and contribute to scientific exploration.
Subsequently, King George Island became a hub for international research, with bases from countries like Russia, China, the United States, and Poland being set up over the following decades. The Polish Arctowski Station (1977) and the Russian Bellingshausen Station (1968) are among the most notable. The Russian station was in October 2018, the site of the first attempted murder in Antarctica.
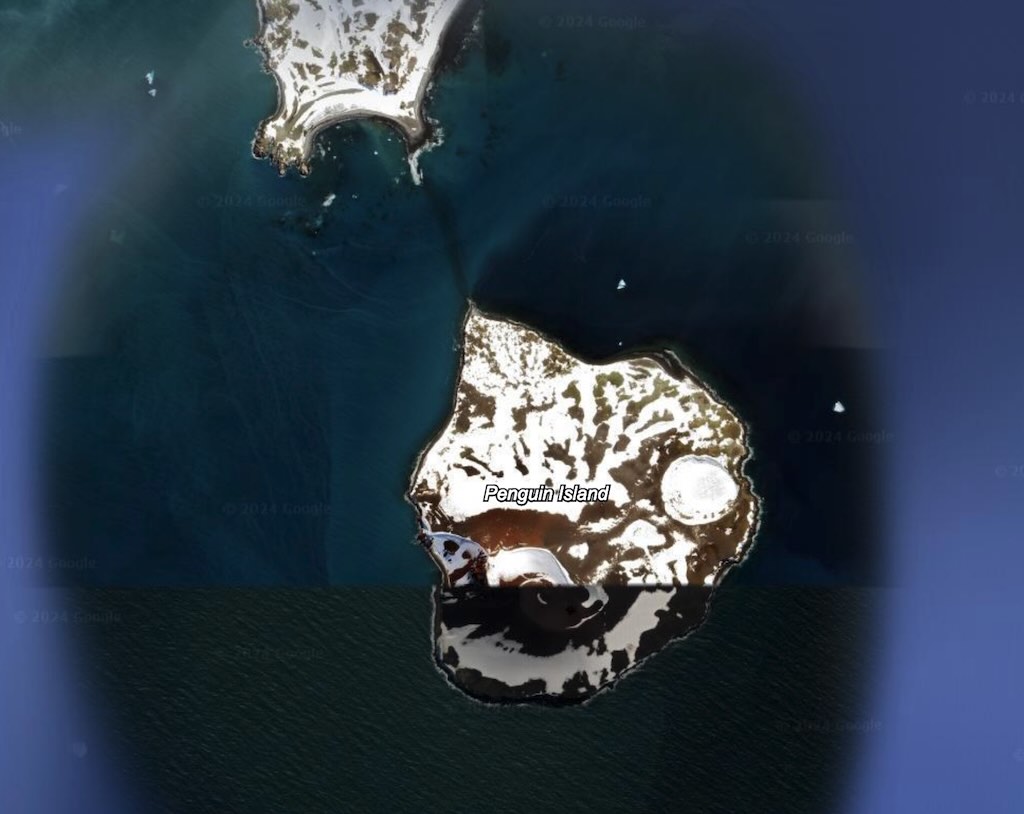
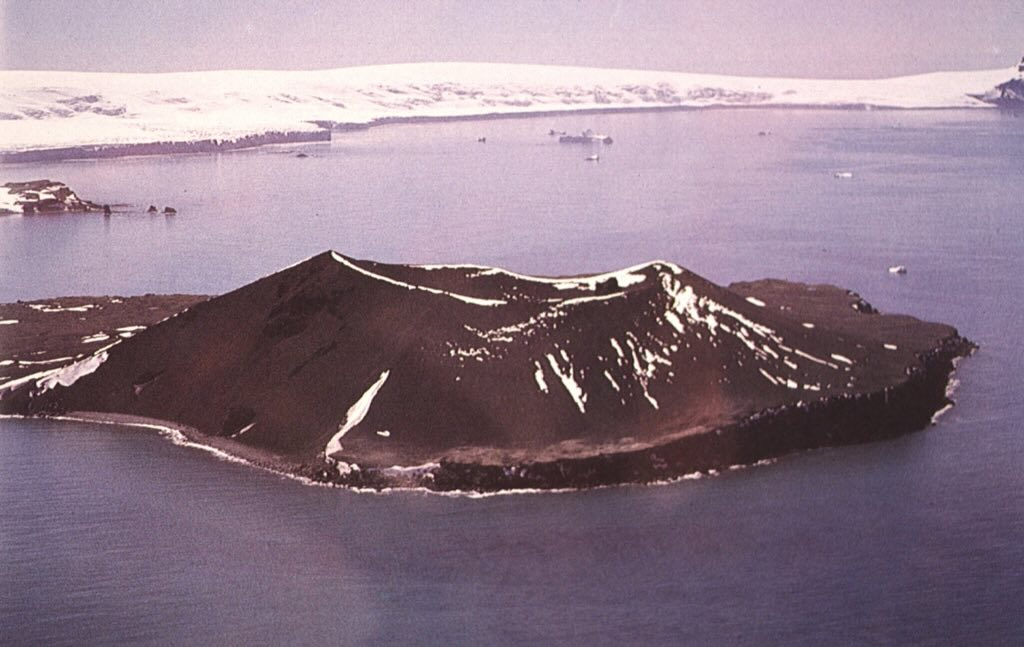
Le Lyrial arrived at Penguin Island in the South Shetland Islands later than expected, and the 7th excursion started almost immediately.
Penguin Island is a small volcanic island that spans approximately 1.7 square kilometres and is characterised by its rugged volcanic terrain, including basaltic lava flows, pyroclastic deposits (rocks ejected during eruptions), and a prominent stratovolcano, Deacon Peak, which rises to 180 meters above sea level. This extinct volcano with its steep profile and summit crater dominates the island’s topography.
The island’s unique geologic composition includes layers of tephra and ash, indicating numerous past eruptions. Penguin Island also hosts a seasonal melting glacier, feeding into its coastal environment.
I think it was the first time I saw so many old bones of whales on the beach. The skeleton of a whale, a marine mammals, provides an insights into their evolutionary history, and which ties them to land-dwelling ancestors.
Whales have a flexible vertebral column divided into 7 cervical vertebrae (the same a humans), 9-17 thoracic vertebrae (depending on species, man has 12), 2-24 lumbar vertebrae (man has 5), sacral (in whales the sacral vertebrae are reduced and not fused as with humans), and caudal regions. Humans typically have 3 to 5 caudal vertebrae, which are fused to form the coccyx (tailbone), whereas whales have 12 to 28, depending on the species.
In whales the cervical (neck) vertebrae are often reduced or fused, limiting head movement but providing streamlining. And the caudal (tail) vertebrae are elongated and support the powerful tail flukes, used for propulsion. Cetacean flukes are horizontal and move up and down, because cetacean spines bend the same way as in other mammals (fish tails are usually vertical and move from side to side).
The vertebrae seen below are quite substantial, and it would take two people to lift one. We can also see one rib. The whales ribcage is more flexible than that of terrestrial mammals, and they lack a sternum. Their ribs are loosely connected, aiding lung collapse during deep dives.
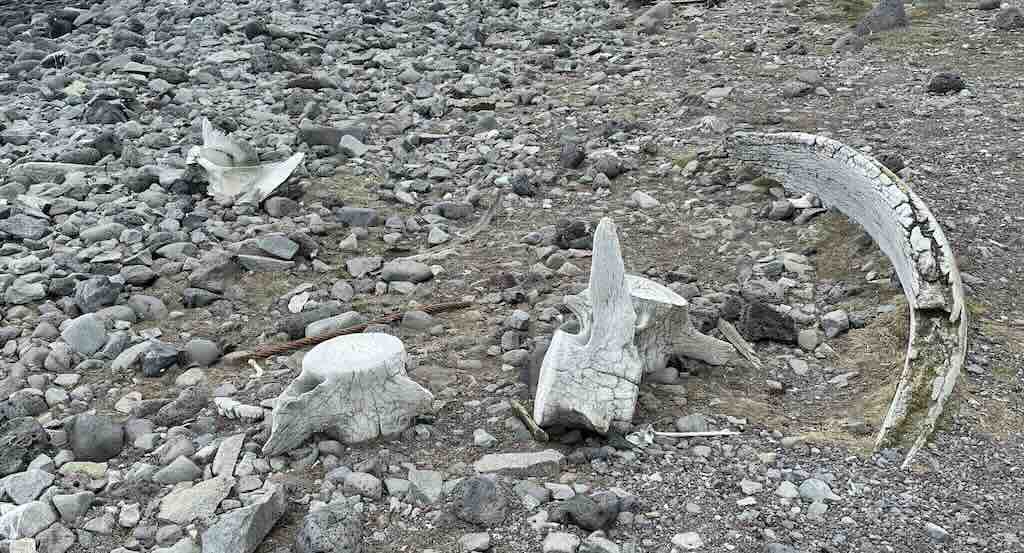
It’s worthwhile remembering that the South Georgia Islands are a British Overseas Territory, and the stringent conservation measures applied there are part of a South Georgia Environmental Management Plan. But this also means that they are not subject of the Antarctic Treaty and the Antarctic Treaty System (ATS). However they are affected by the Convention on the Conservation of Antarctic Marine Living Resources (CCAMLR), part of the ATS, because it indirectly affects the region’s marine resources, as it governs sustainable fishing in the Southern Ocean.
In this second part of the cruise, and with this first excursion on Penguin Island, we were under the Antarctic Treaty, which provides visitor site guides for all the places that permit excursions. They are guides destined for those organising visits, rather than for the visitor themselves.
Each guide lists the topography, fauna and flora found there. It warns about the know and potential impact visitors have, and the landing requirements. It isolates the visiting areas, e.g. indicating the guided walks and if free roaming is permitted (often not). For example (see below), it clearly indicates the landing site and the path than can be taken to visit the crater and cone.
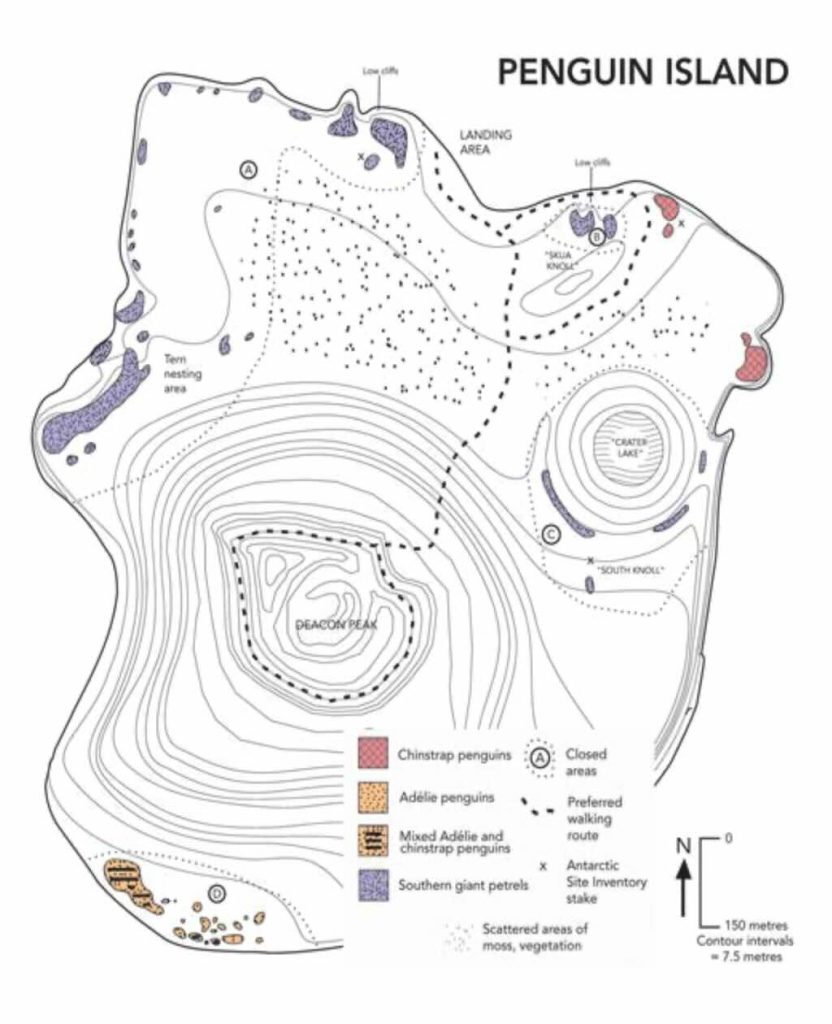
It was interesting to see that had Le Lyrial been fully booked with over 200 passengers, it would not have been allowed to visit Penguin Island. The limit is 200 guests onboard, and only 100 are allowed on the island at any time, and there must be a guide for every 20 visitors on the island. In addition only 2 ship are allowed to visit in any 24 hour period, and not at the same time.
Excursion - Admiralty Bay
Originally the 8th excursion was planned for Turret Point, however, we actually went for a zodiac cruise in Admiralty Bay. No doubt Turret Point would have been interesting, and I don’t know why our second excursion was changed, possibly it was just a problem to find a landing spot. In any case I think everyone found our visit to Admiralty Bay a fantastic new experience.
New for two reasons. For the first time, excluding the hamlet Grytviken on South Georgia, we saw one of the bases dedicated to scientific research. And also for the first time, we saw sea ice close up.
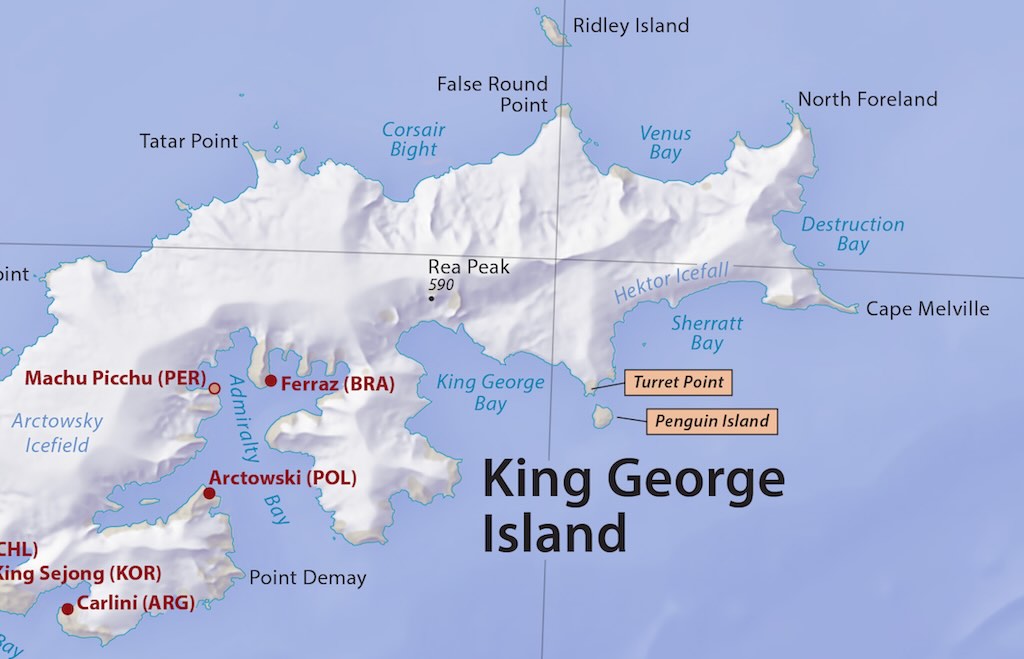
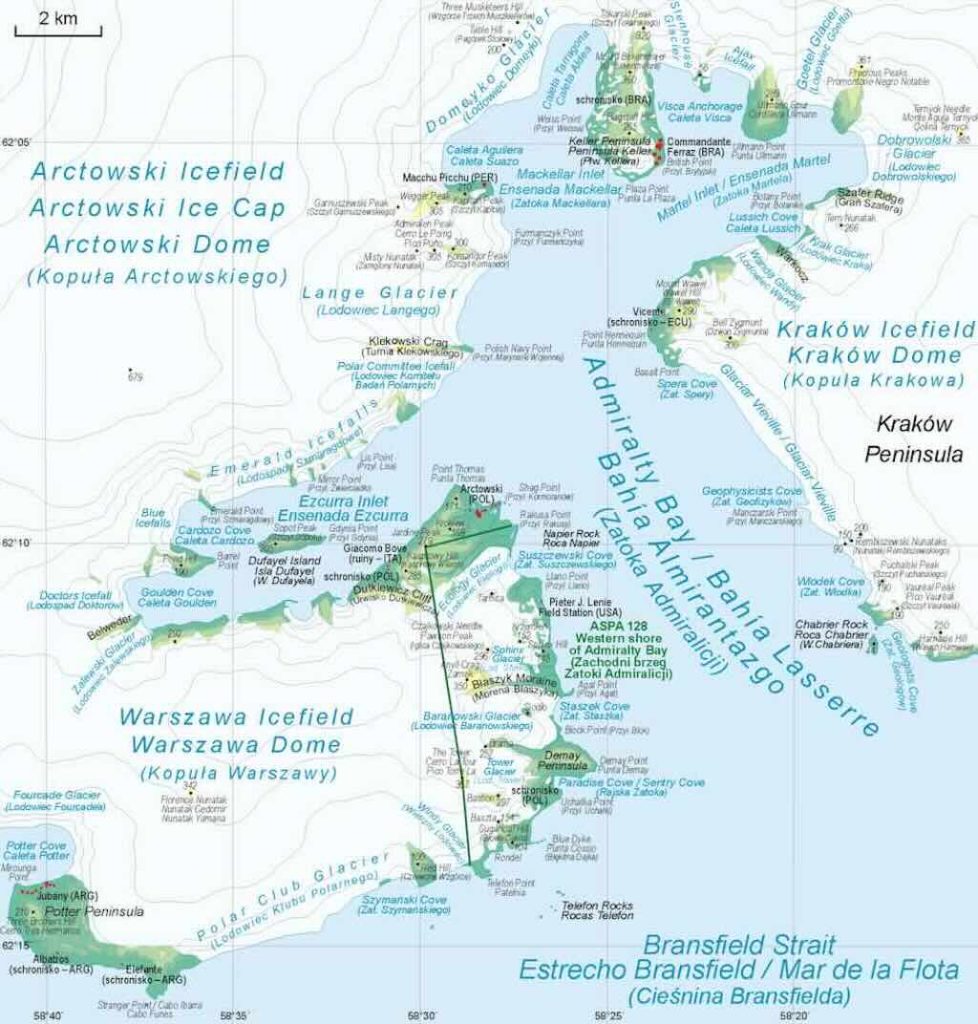
We actually sailed deep into Admiralty Bay, into the Mackellar Inlet, in front of the Domeyko Glacier, and with the Keller Peninsula on our right.
Entering the inlet we found on our left the Estación Científica Antártica “Machu Picchu” (ECAMP), or the Machu Picchu Scientific Base. My understanding is that the base has been there since 1988, and it’s made up of 11 modules. N° 1 and 2 for habitation, 3 for cooking, 4 for supplies, 5 for laboratories, 6 for powerhouses, 7 and 8 for maintenance and vehicle storage, 9 for waste, 10 for shelter and 11 for a new powerhouse. It can accommodate 43 people but is only open during the southern summer.
They have 22 scientific research activities in oceanography, geology, biology, environment, volcanism, hydrography, etc., and on some of them they collaborate with teams from Argentina, Belgium, Chile, Columbia, Ecuador, Germany and Portugal.
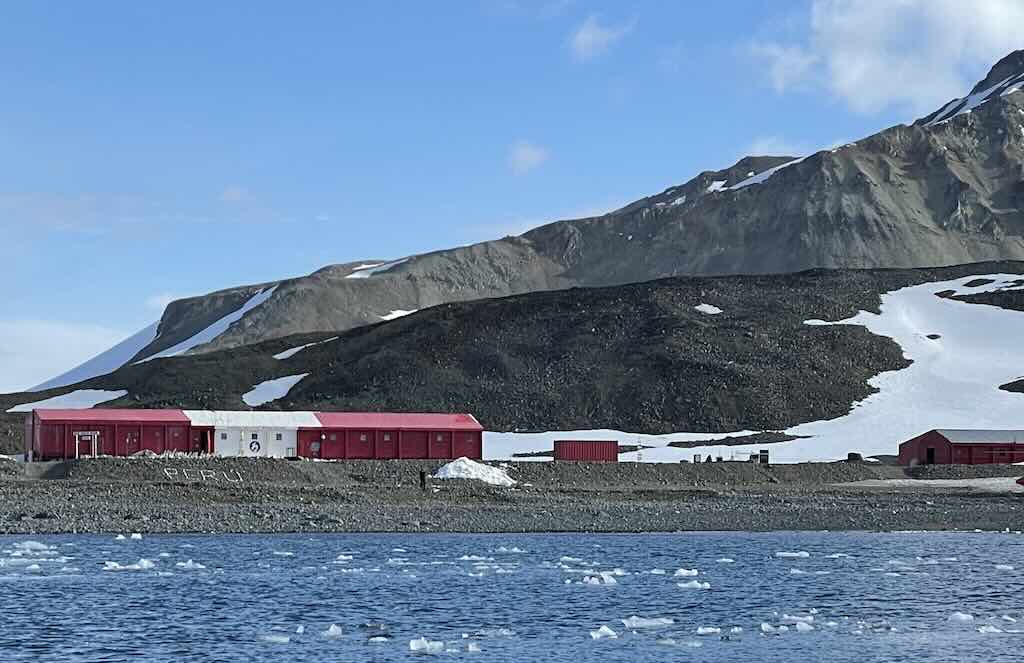
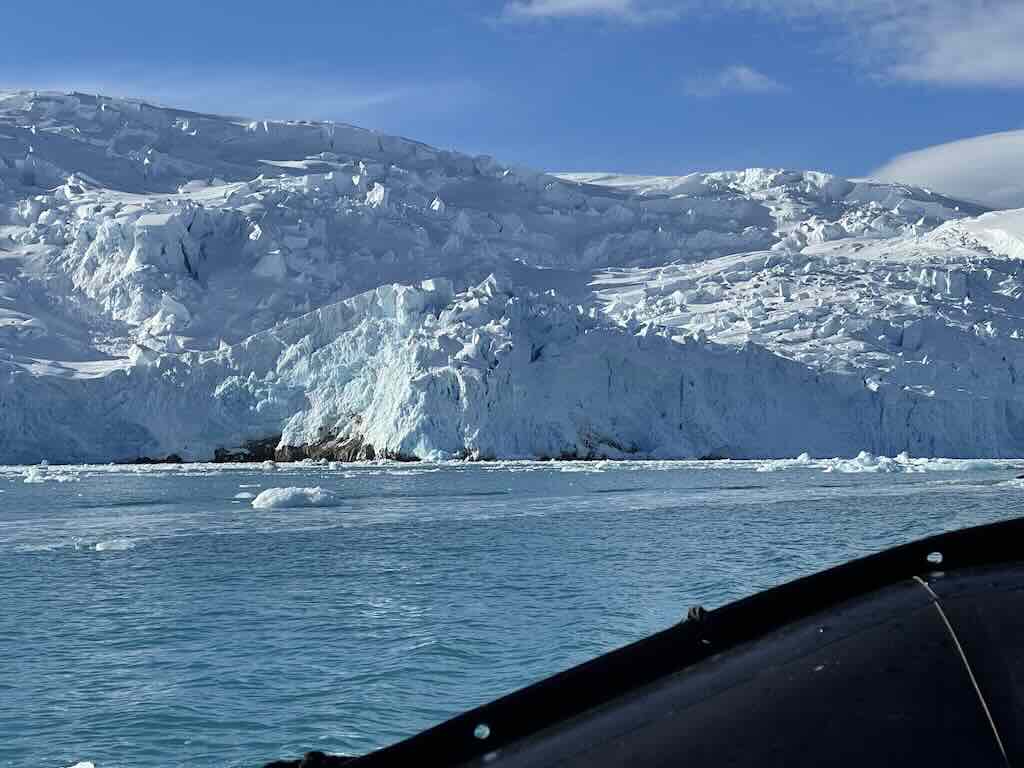
Deeper into the inlet we found the coast of the bay covered in glacial deposits, and there was an abundance of sea ice still present.
19:30 Officers' Dinner
After the daily recap & briefing at 17:45, there was the traditional Officer’s Dinner.
I didn’t take this event very seriously, and ended up booking the last available place. Everyone was supposed to wear white, and follow the dinner with the “White Evening” dance at 21:30. I didn’t wear white and I didn’t dance!
On our table we were lucky to have one of the Engineering Officers and the ships IT Officer. Guests included an English-born South African retired mining engineer who had also lost his wife last year, and two Taiwanese. One was a widow who spoke halting English, and the other was a young man, who spoke an excellent English.
The Engineering Officer was Rumanian, in his early 30s, married, with a strong sense of family, etc. His work involved staffing the bridge in 4-hour shifts, and looking after three of the ships engineering packages, including the water system. He had worked on a larger cruise ship, but preferred the small sized Ponant ships, and liked the Antarctic-Artic routes. He had been able to negotiate a 2-month work/home leave cycle.
The IT Officer was the only one on board, and ran everything from his cabin. He was from the Philippines and was 49 years old. He had worked in electronics hardware, including a period in Taiwan, and only later did he join a large Italian cruise company, before joining Potant. He was working a 4-month work cycle.
He mentioned that the passenger internet connection was provided through two StarLink antenna. This left the classical domes for “real-time, must-have” connections for the bridge, and the StarLinks were for the “nice-to-have” links for passengers.
I took 50 mg dimenhydrinate just before bed.
What's So Special About Sea Ice?
Sea ice is frozen ocean water that forms, grows, and melts in the ocean. Unlike icebergs, which originate from glaciers and are composed of freshwater, sea ice is primarily saline but undergoes desalination as it forms and ages. It plays a critical role in the Earth’s climate, ocean circulation, and ecosystem.
The formation of sea ice begins when the surface of seawater cools below its freezing point, which is approximately -1.8°C at typical ocean salinity (~35 PSU, practical salinity units). As the water cools, frazil ice (small needle-like ice crystals) forms and coalesces into larger ice masses.
Stages of sea ice formation
- Frazil Ice: Tiny, suspended ice crystals (0.1 to 3 mm in diameter) form in supercooled water.
- Grease Ice: A thin, soupy layer of loose ice crystals accumulates on the surface.
- Nilas Ice: A thin, elastic layer of ice (up to 10 cm thick) forms, which bends without breaking. Nilas can be further subdivided into dark nilas (up to 5 cm (2.0 in) in thickness and very dark) and light nilas (over 5 cm (2.0 in) in thickness and lighter in colour).
- Pancake Ice: Circular, slushy ice floes with raised edges form in turbulent waters. This is a kind of ice that in the open ocean may damage the hulls of ships.
- Young Ice: Ice thickens beyond 10 cm and can start to develop brine channels. Young ice can be further subdivided into grey ice (10-15 cm in thickness) and grey-white ice 15-30 cm in thickness). Under compression young ice will either raft (at the grey ice stage) or ridge (at the grey-white ice stage).
- First-Year Ice: Ice that has survived at least one winter, reaching thicknesses of 30 cm to 2 m. First-year ice may be further divided into thin (30-70 cm), medium (70-120 cm) and thick (>120 cm).
- Multiyear Ice: Ice that has persisted for multiple seasons, losing salinity and increasing in strength and hardness. Old ice is commonly divided into two types, second-year ice, which has survived one melting season and multiyear ice, which has survived more than one year.
There exists also rotten ice, a loose term for ice that is melting or structurally disintegrating due to being honeycombed by liquid water, air, or contaminants trapped between the initial growth of ice crystals. Rotten ice has a subtype called candle ice, which has a columnar structure. Like other rotten ice, it poses a hazard due to its lack of structure.
Structure and properties
Sea ice is characterized by a porous, layered structure due to the presence of brine inclusions, air pockets, and salt rejection processes during freezing. Some key properties include:
- Salinity & Brine Rejection: Freshly formed sea ice contains brine pockets with salinity up to 100 PSU, but over time, gravity drainage expels brine, reducing the salinity to about 4-7 PSU in multiyear ice.
- Thermal Conductivity: Varies depending on age and structure; young ice has lower thermal conductivity (~1.2 W/m·K) compared to older ice (~2.0 W/m·K).
- Albedo (Reflectivity): Fresh snow-covered ice has an albedo of 80-90%, whereas melting ice has a lower albedo (~50%), influencing Earth’s heat balance.
- Mechanical Strength: Sea ice is relatively brittle but can deform under stress, forming pressure ridges and leads (cracks).
- Density: Ranges from 850 to 910 kg/m³, making it less dense than seawater (~1025 kg/m³), which is why it floats.
Special characteristics of sea ice
- Brine Channels & Permeability: Unlike freshwater ice, sea ice retains small pockets of brine, creating a dynamic microstructure. These channels facilitate microbial habitats and influence ice mechanics.
- Ice-Ocean Interaction: Sea ice insulates the ocean, preventing excessive heat loss and reducing evaporation. It also contributes to thermohaline circulation by rejecting salt during freezing, increasing water density and driving deep-ocean currents.
- Seasonal Dynamics & Thickness Variability: Arctic sea ice exhibits perennial (multiyear) characteristics, while Antarctic sea ice is largely seasonal due to differing oceanic and atmospheric conditions.
- Impact on Climate: The ice-albedo feedback mechanism amplifies climate change effects; as sea ice melts, darker ocean water absorbs more heat, leading to further ice loss.
We have only scratched the surface of sea ice. When sea ice forms, it expels salt, increasing the density of the underlying seawater. This dense, salty water sinks, driving global ocean currents (especially the thermohaline circulation). So sea ice formation influences deep-ocean circulation, affecting climate on a planetary scale.
Brinicles, are large, hollow ice stalactites that form underwater beneath sea ice. They are rare, but are sometimes called “ice fingers of death”, because they can trap and kill slow-moving sea creatures like starfish and urchins.
And Frost Flowers, are delicate, ice-crystal formations that grow on the surface of newly formed sea ice. They resemble white, feathery flowers. but can be up to three times saltier than seawater.
Finger Rafting, is when thin ice sheets collide at shallow angles, they interlock in a zig-zag ‘fingering’ pattern rather than simply stacking. Unlike other ice that fractures upon collision, sea ice rafts deform in unique ways.
When sea ice is pushed together by winds or ocean currents, it fractures, folds, and stacks. This can create Ice Hummocks, which are small hills or irregular mounds of ice formed by compression. If the pressure increases it can create Pressure Ridges, massive, jagged ice structures formed when sheets of ice collide and buckle. Some ridges can reach up to 10 meters thick.
Even when air temperatures are below freezing, sea ice can melt from underneath due to warmer ocean currents. This leads to Basal Melting, a process where sea ice thins from below while appearing stable from above.
Generally Antarctic sea ice is not enclosed, and can expand freely, leading to greater ice divergence (spread) and thinner ice. The absence of land barriers means Antarctic sea ice does not pile up into thick ridges as often as in the Arctic, resulting in a thinner, more mobile ice pack. Antarctic sea ice is generally 1–2 meters thick, whereas Arctic sea ice (especially multiyear ice) can be 3–4 meters thick. Due to frequent snowfall, Antarctic sea ice tends to accumulate a thicker layer of snow, but the weight of accumulated snow can push thin ice below the waterline, allowing seawater to flood the snow layer. This leads to the formation of snow ice, a process less common in the Arctic.
Because Antarctic sea ice forms and melts seasonally, it retains more brine than the Arctic’s older ice. However, Antarctic sea ice contributes to the formation of Antarctic Bottom Water (AABW) by releasing brine during freezing, increasing ocean density and driving deep-sea currents.
Antarctic sea ice is often covered with snow, which can insulate the ice and reduce its melting rate, but also lower its reflectivity in some cases. And since Antarctic sea ice fluctuates seasonally, it plays a major role in modulating global heat exchange, particularly through the formation of cold, dense water that influences ocean circulation.
The seasonal melt of Antarctic sea ice releases nutrients, driving massive phytoplankton blooms that support the Southern Ocean food web. Antarctic krill populations are highly dependent on sea ice, as their larvae feed on ice algae growing within the brine channels.
How Do Whales Navigate In Ice-covered Regions?
During the cruise we saw a few whales, and I wondered how they deal with things like sea ice.
It would appear that sea ice affects the whales ability to navigate under and around it. It affects sound transmission, breathing opportunities, and migration routes. Since whales rely heavily on echolocation and passive listening, they must adapt their behaviour in ice-covered waters.
Firstly, sea ice affects sound transmission, since thin ice transmits sound more efficiently than open water, and can act like a reflective ceiling, allowing sounds to travel farther. But thick ice and pressure ridges absorb and scatter sound waves, making long-distance communication harder. They can even create acoustic shadows, where sound is blocked. Some species, like bowhead whales, have evolved to produce lower-frequency calls that travel farther under ice. Otherwise whales tend to increase call intensity (louder vocalisations) to overcome sound loss.
Background noise from shifting ice can make it harder for whales to hear each other, as can human activities (ships, icebreakers, underwater seismic surveys) which use low-frequency noise. Whales change their vocalisation frequency to avoid background noise. Also some species (like belugas) use high-frequency echolocation clicks, which are less affected by ice noise.
On the other hand sea ice can act as a shield against predators such as killer whales (orcas), which prefer open water. Narwhals and belugas often retreat under thick ice, where orcas have difficulty manoeuvring. For example, narwhals can hold their breath for up to 25 minutes to avoid being seen.
What is "Iceberg Alley" and Nautical Twilight?
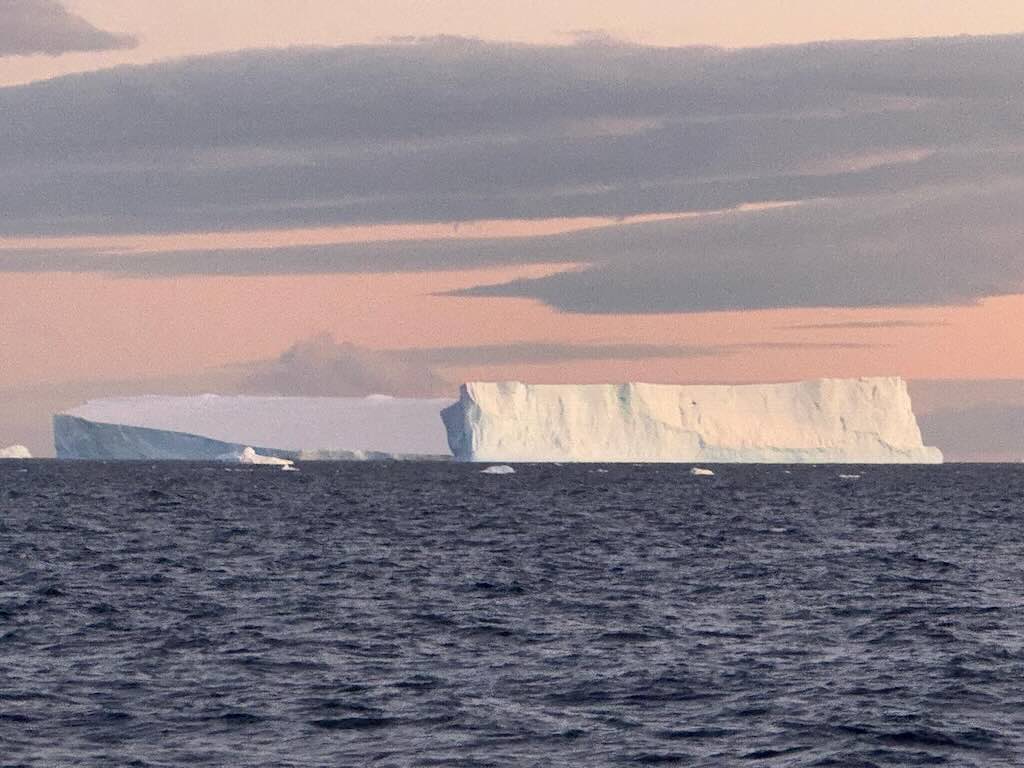
“Iceberg Alley” is a term used to describe regions where icebergs are frequently encountered due to ocean currents and glacial activity. In the context of the South Shetland Islands, this term refers to the waters surrounding the archipelago, particularly the Antarctic Sound, which separates the tip of the Antarctic Peninsula from Dundee Island. This area is colloquially known as “Iceberg Alley” because of the numerous and often massive icebergs that drift through these waters.
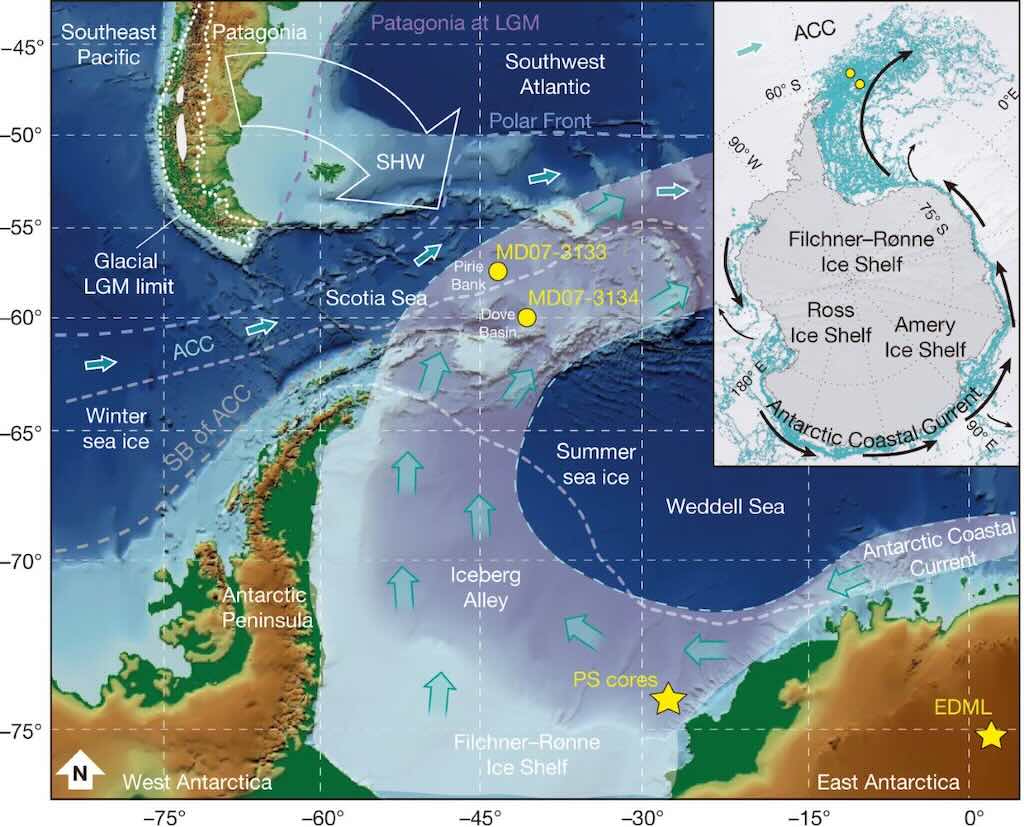
I’ve highjacked a graphic, so we can ignore all the abbreviations, etc. What we see is the Antarctic Iceberg Alley that carries icebergs across the tip of the Antarctic Peninsula and the northern part of the South Shetland Islands.
The South Shetland Islands are located about 120 kilometres north of the Antarctic Peninsula, encompassing 11 major islands and several minor ones, with a total land area of approximately 3,687 square kilometres. The region is characterised by a harsh climate, with strong westerly winds and temperatures often remaining below freezing for most of the year. Over 80% of the islands’ terrain is permanently glaciated, contributing to the frequent calving of icebergs into the surrounding seas.
The moniker “Iceberg Alley” is also applied to other regions, notably the waters off the coast of Newfoundland, Canada. In this area, icebergs calved from Greenland’s glaciers drift southward, posing significant hazards to maritime activities. This is the region where the RMS Titanic tragically sank in 1912 after colliding with an iceberg.
The prevalence of icebergs in these regions is primarily due to ocean currents. In Antarctica, the Antarctic Coastal Current circulates icebergs around the continent until they reach the Weddell Sea. There, the flow is deflected northward by the Antarctic Peninsula, funneling icebergs through the area known as “Iceberg Alley” before they enter the warmer waters of the Antarctic Circumpolar Current, where they eventually melt.
Navigating through “Iceberg Alley” is perilous due to several factors:-
Unpredictable Iceberg Movements: As icebergs drift into warmer waters, they melt and can become unstable, leading to unexpected shifts or rotations. This unpredictability poses significant risks to vessels, as the submerged portions of icebergs can cause collisions even if the visible part appears at a safe distance.
Size and Variety of Icebergs: The region hosts a diverse range of iceberg sizes and shapes, from small fragments to massive tabular icebergs spanning several kilometers. The sheer size of some icebergs can obstruct navigation routes, while smaller, less visible pieces, known as “growlers” or “bergy bits”, can still inflict substantial damage upon impact.
Harsh Environmental Conditions: The South Shetland Islands experience strong westerly winds, frequent storms, and low visibility due to fog and snowfall. These conditions, combined with the presence of icebergs, make navigation extremely challenging and hazardous.
In summary, “Iceberg Alley” in the vicinity of the South Shetland Islands is a region marked by the frequent presence of icebergs due to glacial calving and ocean currents.
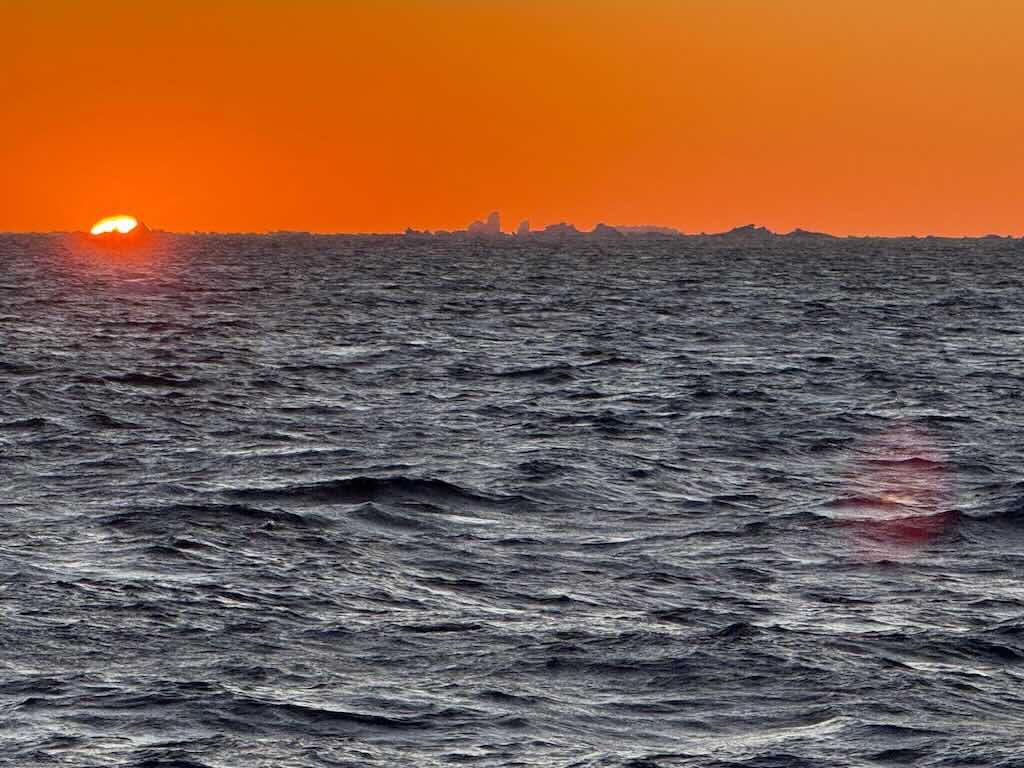
Between December 5-6, 2024, as we journeyed from Admiralty Bay to Brown Bluff in Antarctica, our night was shaped by the unique polar environment of the austral summer.
Lighting conditions:
During early December, the Antarctic region experiences continuous daylight or extended twilight due to the Earth’s axial tilt. Between 22:19 and 02:40, the sun was below the horizon, but the sky was not completely dark. This phenomena is known as nautical twilight, where the sun is between 6 and 12 degrees below the horizon, providing a faint illumination. This usually results in a dimly lit environment with a deep blue sky, allowing for general outdoor visibility without artificial lighting.
Sunset and twilight:
In this region, the sun descends gradually, creating prolonged periods of twilight. The setting sun casts a warm, reddish-orange hue across the horizon, a spectacle often referred to as the “Antarctic sunset glow“. This extended twilight can last for several hours, with the sky transitioning through various shades of red and purple before reaching the deeper blues of nautical twilight. However,
At Admiralty Bay (~62°S) and Brown Bluff (~63.5°S), the Sun never fully sets by December. The Sun dips just below the horizon, but the sky remains illuminated, and this extended twilight can last for several weeks, spanning roughly late November to early January.
In early December the sun descends towards the horizon, initiating a prolonged sunset. As the sun approaches the horizon, its light traverses a greater thickness of the Earth’s atmosphere. This increased path length in air causes shorter blue/violet wavelengths to scatter out of the line of sight, while longer red/orange wavelengths dominate, resulting in a vivid red sky. This is a phenomenon known as Rayleigh scattering. The presence of atmospheric particles, such as ice crystals and aerosols, can enhance this effect, scattering more blue and green light, and intensifying even more the red hues observed during sunset. In addition, in polar regions, where the Sun moves more horizontally than vertically, refraction extends twilight periods, allowing the sky to remain illuminated for hours after the Sun has technically set. This bending effect can also make the Sun appear flattened or distorted near the horizon.
6 December 2024 - Brown Bluff and Kinnes Cove (Day 11)
Calm night, woke around 04:30 local time.
Temperature -1 to -8°C and a 2-24km/h wind during the day.
Sunrise was at 02:40 and sunset at 22:38.
The day started with breakfast at 07:00, and having decided that I would always avoid the morning stretching, I will no longer mention it. I also avoided the morning beginners salsa lesson.
Excursion - Brown Bluff
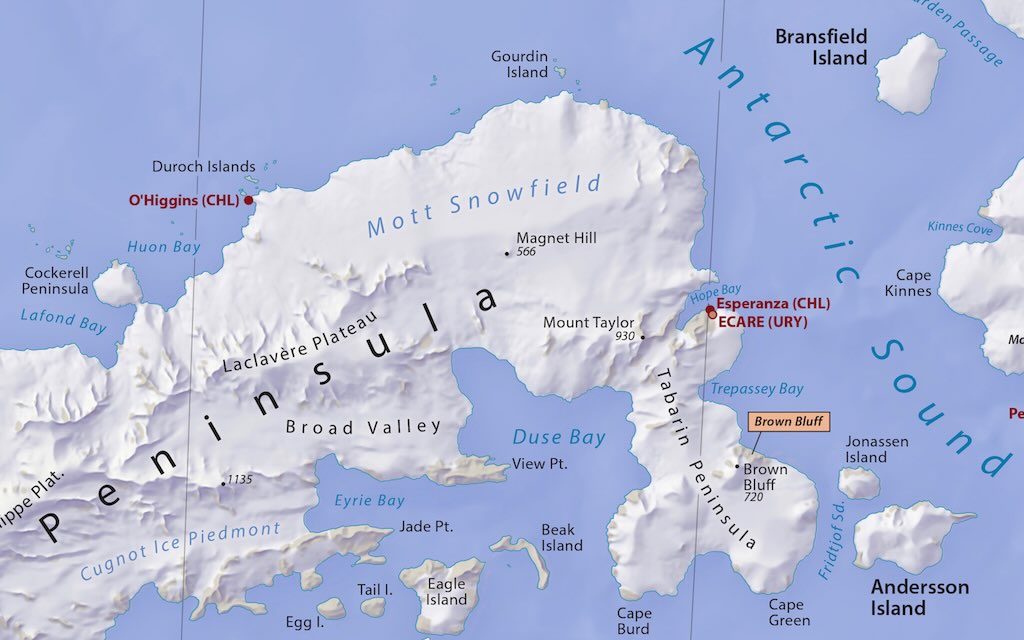
Overnight we had moved from the South Shetland Islands to Brown Bluff actually situated on the Antarctic Peninsula.
So finally we would set foot on mainland Antarctica. The Antarctic Peninsula is the northernmost extension of the Antarctic continent, projecting toward South America. While it is connected to the main body of Antarctica, it is distinct due to its mountainous terrain and relatively milder climate compared to other parts of the continent. Its unique location makes it one of the most biologically active and accessible regions of Antarctica.
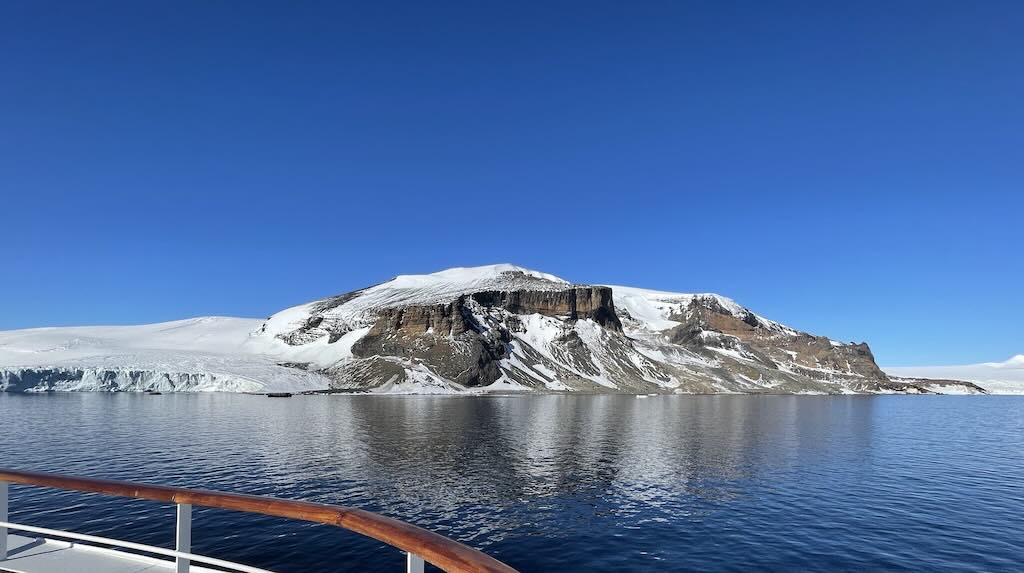
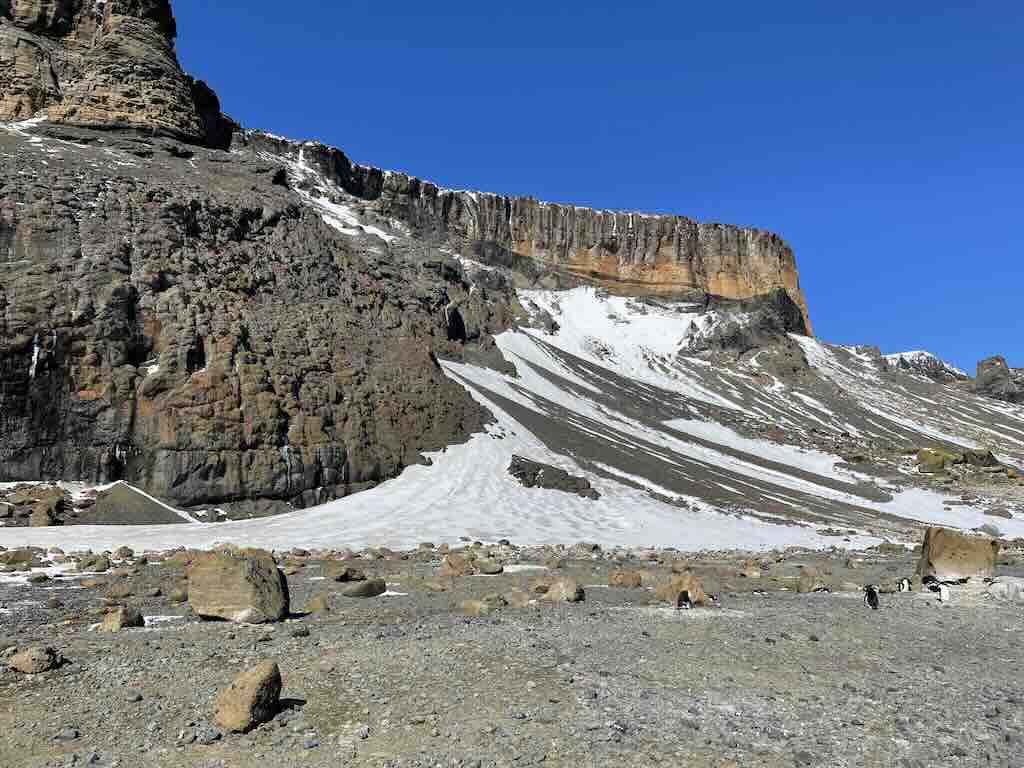
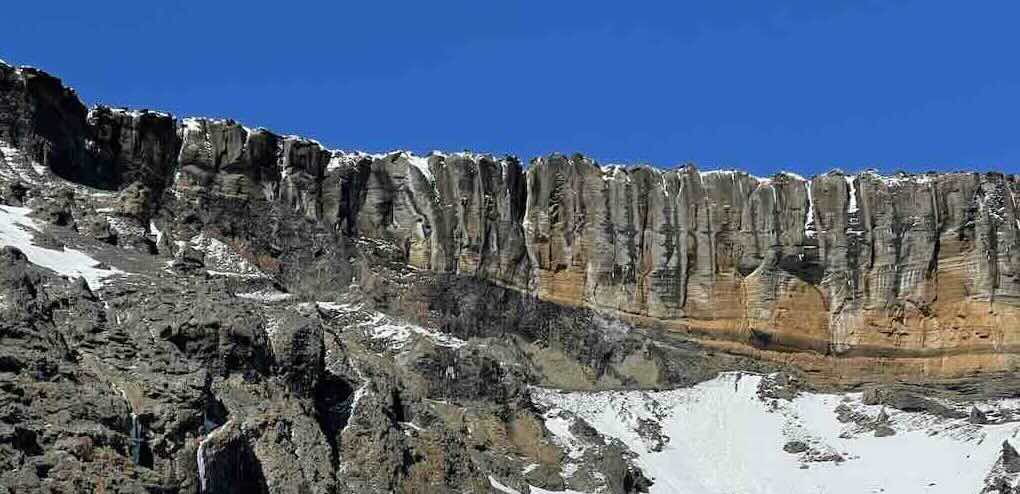
What Can Brown Bluff Tell Us About Volcanoes?
Brown Bluff is located on the Tabarin Peninsula, which forms the east extremity of Trinity Peninsula in the Antarctic Peninsula. It is a geologically significant site formed by volcanic activity approximately one million years ago. This formation is classified as a tuya, a type of volcano that would have erupted beneath an ice sheet or glacier, resulting in a flat-topped, steep-sided structure. The unique geology of Brown Bluff provides valuable insights into subglacial volcanic processes and the region’s glacial history.
Geological formation
The formation of Brown Bluff occurred during the Pleistocene epoch, between 2.58 million to 11,700 years ago, spanning the Earth’s most recent period of repeated glaciations. Often volcanic eruptions took place beneath a thick ice sheet, in this case it’s estimated to have been over 400 meters thick. These subglacial eruptions led to the accumulation of volcanic materials within an englacial lake, creating distinct geological features. The structure of Brown Bluff can be divided into several stages:
Pillow Volcano Stage: Initial eruptions under high-pressure conditions formed pillow lava (bulbous, pillow-shaped structures typically created when lava extrudes into water).
Tuff Cone Stage: As volcanic activity continued, explosive interactions between magma and meltwater produced fine-grained volcanic ash, which settled to form a tuff cone.
Slope Failure Stage: Structural instability led to collapses and landslides, reshaping the volcanic edifice.
Hyaloclastite Delta Stage: The final stage involved the formation of hyaloclastite, a glassy, fragmented material resulting from rapid cooling of lava in water, deposited as deltas.
These stages collectively contributed to the current morphology of Brown Bluff, characterised by its steep cliffs composed of volcanic breccia and tuff. The reddish-brown coloration of the cliffs is due to the oxidation of iron-rich minerals within the volcanic rocks.
The name “Brown Bluff” was assigned by the Falkland Islands Dependencies Survey (FIDS) following their survey in 1946. The designation reflects the prominent cliff of reddish-brown volcanic rock on the mountain’s northern face.
Beyond its geological importance, Brown Bluff serves as a vital ecological habitat. The area supports a breeding colony of approximately 20,000 pairs of Adélie penguins and around 550 pairs of Gentoo penguins. Other avian species, such as Cape petrels, Wilson’s storm petrels, and Kelp gulls, also nest in the region. Marine mammals, including Weddell seals and Leopard seals, are frequently observed along the shoreline and in the surrounding waters.
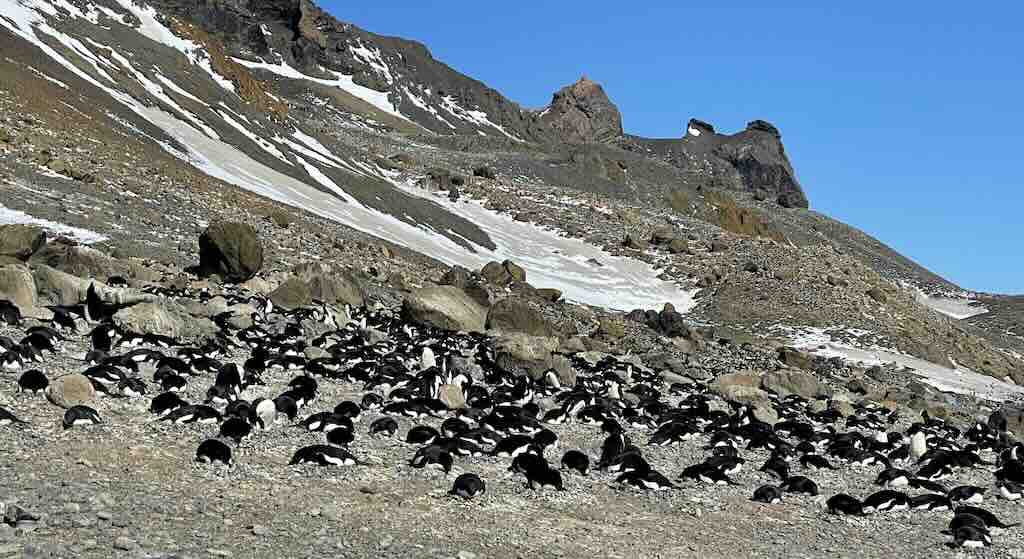
The area is a prime wildlife spot, home to large colonies of Adélie and Gentoo penguins. Its ice-strewn beach, coupled with stunning views of glaciers and icebergs, makes Brown Bluff a popular destination for Antarctic expeditions.
Reclassification of Gentoo Penguins
Genetic and morphological studies suggest that gentoo penguins, previously considered a single species, should be classified into four distinct species. This research highlights differences in their physical traits and genetic makeup, driven by geographical isolation over time. Such reclassification could enhance conservation strategies by allowing more precise monitoring of population trends and responses to environmental changes.
How Do Adélie and Gentoo Cohabit on Brown Bluff?
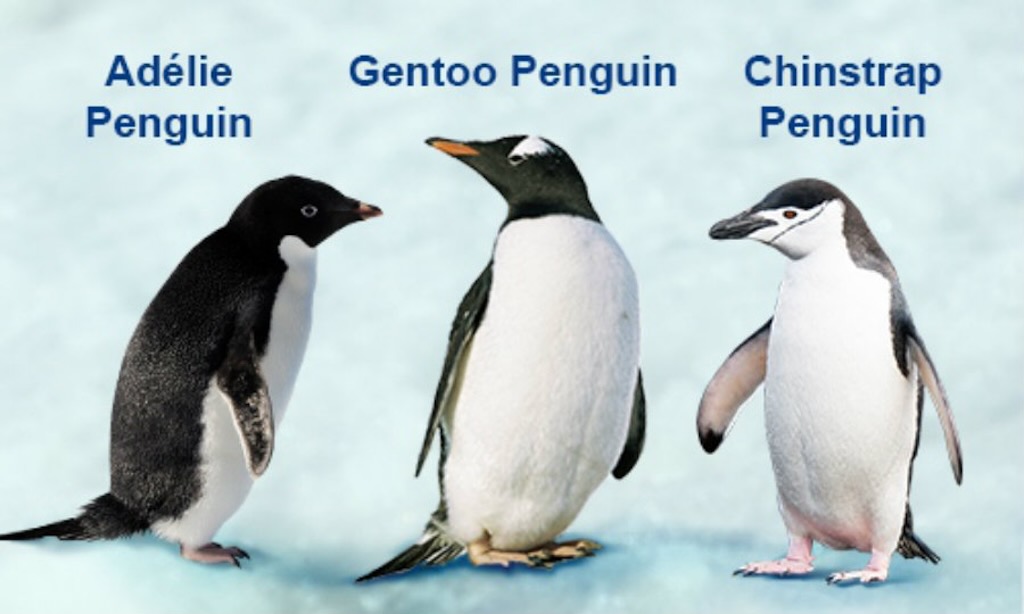
Brown Bluff is a unique location where both Adélie penguins (Pygoscelis adeliae) and Gentoo penguins (Pygoscelis papua) coexist. However, despite sharing the same habitat, they have distinct behaviours, appearances, and interactions.
How to tell them apart?
Adélie penguins are smaller, about 46-71 cm tall and weighing 3.6-6 kg. The most prominent feature is the white eye ring, and they have a classic black-and-white tuxedo appearance. They have longer tail feathers than gentoos, and are more upright and stiff-legged.
Gentoo Penguins are larger, about 51-90 cm tall, weighing 4.5-8.5 kg. They don’t have a distinctive white eye ring , but have a black head with a wide white stripe extending like a cap from one eye to the other. The have a shorter tail, and are less stiff and waddling more.
When reading around the subject of penguins, you encounter a significant oversimplification in some sources that claim penguins are solitary animals. This often stems from a misunderstanding of their behaviour.
While penguins do spend time alone, especially during foraging trips or molting seasons, the overwhelming majority of species are highly social, colonial birds.
Where does the “Solitary Penguin” myth come from?
- Foraging Behavior: Penguins spend long hours or days at sea alone while hunting, leading to the impression that they prefer solitude.
- Molting Period: When penguins molt, they isolate themselves for 2–4 weeks since they cannot swim and must conserve energy.
- Territorial Disputes: Some species, especially Adélie and Chinstrap penguins, are extremely territorial, leading to frequent aggressive interactions, which might seem like a preference for solitude.
- Misinterpretation of Antarctic Footage: Many documentaries focus on a single penguin navigating ice or facing hardship, reinforcing the lone-survivor narrative.
- Some Species Are Less Social: Unlike Emperor penguins (which form tight huddles), species like the Galápagos or Yellow-eyed penguins are less colonial and often breed in small, scattered groups.
The reality is that penguins are among the most social birds.
Penguins depend on their colonies for:
- Thermoregulation (especially emperor penguins).
- Nesting security (protection in numbers).
- Chick-rearing (parents recognise chicks through vocal calls in massive groups).
- Courtship and lifelong partnerships (many species return to the same mate yearly).
Even when penguins forage alone, they still rely on social structures when they return to land.
Contradictory claims & context
- It’s true that most species of penguins are highly social and form dense colonies.
- Penguins hunt alone and do not interact at sea, but they often leave the colony together and return in waves.
- It’s true that some species of penguins are aggressive and territorial, and don’t cooperative, but they still form massive nesting colonies.
- It is true that some penguins spend months at sea alone, but are entirely dependent on colony life during winter.
Being social, does not mean always being friendly
- Penguins are not solitary, but they are not always cooperative either.
- They form dense colonies, but within those colonies, rivalries and conflicts are common.
- They often forage alone, but some species (e.g., Emperors and Rockhopper penguins) coordinate their hunting efforts.
So do penguins have friends?
Yes! Penguins form strong social bonds:
- Mating Pairs: Many species are monogamous for a season, and some, like Gentoo penguins, reunite with the same mate every year.
- Friendships: Some penguins form long-term bonds with certain individuals, cooperating for protection or preening each other.
- Colony Recognition: Even in massive colonies of thousands, penguins recognize their mate and chick by voice alone.
Some key differences
Despite sharing the same location, these species have evolved to minimise direct competition by differentiating their habits.
Nesting habits & territory
- Adélie Penguins:
- Prefer flat or gently sloping areas with lots of pebbles for nesting.
- Highly territorial, and will aggressively defend their nest from intruders.
- Form tight, dense colonies with high social interaction.
- ‘Pebble Theft’ they are known for stealing pebbles from each other’s nests, a critical part of their breeding success.
- Gentoo Penguins:
- Nest slightly higher on rocky outcrops or in areas with less snow accumulation.
- More spaced out nests compared to Adélies.
- Less aggressive about nesting materials but still competitive.
Breeding & raising chicks:
- Adélie Penguins:
- Lay two eggs, both parents take turns incubating for ~35 days.
- Highly synchronized breeding cycles (colonies lay eggs within a short period).
- Chicks form crèches (nursery groups) for safety.
- Depart early in the season, as they migrate northward to follow shifting sea ice.
- Gentoo Penguins:
- Lay two eggs, incubate for 34-37 days.
- Less synchronised breeding—some pairs breed earlier or later than others.
- Chicks also form crèches but stay longer in the colony.
- Non-migratory—stay in the region year-round.
They cohabitation without “getting along”
Adélies and Gentoos live in close proximity but tend to avoid one another. Their interactions are mostly neutral, but tension can arise.
Why they don’t get along:
- Competition for Nesting Sites: While Adélies prefer lower areas and gentoos nest higher up, there is some overlap.
- Different Levels of Aggression: Adélies are more aggressive and will chase Gentoos away if they get too close.
- Food Rivalry: Both eat krill, squid, and small fish, so competition is inevitable, but Gentoos dive deeper (~200m vs. ~50m for Adélies), which reduces direct food competition.
Do they ever reproduce together?
- No, they do not interbreed, they are different species with distinct genetics and breeding behaviours.
- Even though they sometimes nest in overlapping areas, they recognise their own species through vocal calls, displays, and mating behaviours.
- No known cases of hybridisation have been observed.
Daily routines & feeding habits
Despite their differences, both species follow structured daily routines.
Adélie Penguins:
- Morning: Depart colony in large groups to feed in coastal waters, targeting krill.
- Midday: Some return to feed chicks, others rest or engage in social behaviors.
- Evening: Return en masse, causing noisy gatherings at the colony.
- Defense: Often chase away intruders, whether they are Adélie neighbours or encroaching gentoo penguins.
Gentoo Penguins:
- Morning: Some leave early, while others linger—less synchronized than Adélies.
- Midday: Take multiple shorter foraging trips; deeper divers than Adélies.
- Evening: Return more gradually rather than in large flocks.
- Defense: More relaxed but still defend their nests from other Gentoos and the occasional Adélie penguin.
Social hierarchy & communication
Penguin communication involves a combination of vocalizations, body movements, and displays, which vary between species and serve different purposes such as mate attraction, territory defense, and chick recognition.
Vocalisations (calls)
Penguins have distinct vocal repertoires, and their calls can generally be grouped into three main types:
- Contact Calls – Used to maintain group cohesion, especially in dense colonies. Example: Adélie penguins emit short, braying calls to stay in touch with their mate or chick.
- Display Calls – Used in courtship, mate recognition, and territory defence. Example: Gentoo penguins perform a trumpeting call, stretching their necks upward while calling loudly to advertise territory or attract a mate.
- Begging Calls – Used by chicks to solicit food from parents. Example: Penguin chicks have distinct, high-pitched calls that allow parents to recognise them in crowded colonies.
Body movements & displays
Penguins rely on physical displays to communicate dominance, courtship readiness, or warnings. Some key movements include:
Ecstatic Display (Trumpeting) – A courtship or territorial call where the penguin stretches its neck upward, flaps its flippers, and emits a loud call. Seen in Gentoos, Adélies, and Emperor penguins.
Bowing – A sign of bond strengthening between mates or a submissive gesture. Common in Gentoo and Adélie penguins, where partners bow before each other to reinforce their bond.
Sideways Gaping & Bill Jabbing -Aggressive display used in territorial disputes. Penguins open their beaks wide, sometimes jabbing at an opponent or intruder.
Preening & Beak Tapping – Used in pair bonding, where mates preen each other’s feathers or gently tap beaks.
Stone Presenting (Courtship Ritual) – Gentoo penguins often present a pebble to a potential mate as part of their bonding ritual.
Chick recognition & parental communication
- Penguins nest in noisy colonies, so parents recognize their chicks by unique vocal signatures rather than sight.
- When returning from feeding, parents and chicks engage in a back-and-forth call exchange until they locate each other.
So why do both Adélie and Gentoo penguins nest in close proximity at Brown Bluff?
There are several factors that might explain this:
Limited suitable nesting sites
- Brown Bluff provides ice-free, rocky terrain, which is crucial for both species’ nesting. Since suitable nesting sites in Antarctica are scarce and competition is high, both species may tolerate each other to take advantage of the available space.
Microclimate and protection
- The north-facing cliffs of Brown Bluff offer some shelter from the harsh Antarctic winds, making it a more favorable microhabitat.
- Proximity to cliffs might also offer protection from skuas, which prey on penguin eggs and chicks.
Differences in nesting preferences and timing
- While both species prefer rocky terrain, Gentoo penguins tend to nest slightly higher on slopes, whereas Adélie penguins often nest closer to the shoreline.
- Their breeding seasons overlap but are slightly staggered, which can reduce direct competition.
- Gentoos tend to start breeding a little earlier, giving them a slight advantage in securing preferred spots.
Food availability and colony stability
- Brown Bluff provides good access to the Weddell Sea, where both species can find ample food (krill, fish, and squid).
- Colonies with multiple species can sometimes be more stable in the face of environmental changes—if one species struggles in a given year, the other may still do well, maintaining overall colony viability.
Social tolerance and colony dynamics
- Some penguin species do not always compete aggressively if food is abundant.
- While Adélie’s can be aggressive, Gentoos are known for being more flexible and adaptable, which might allow them to nest near a more territorial species without major conflict.
How Many Penguins Did You Say?
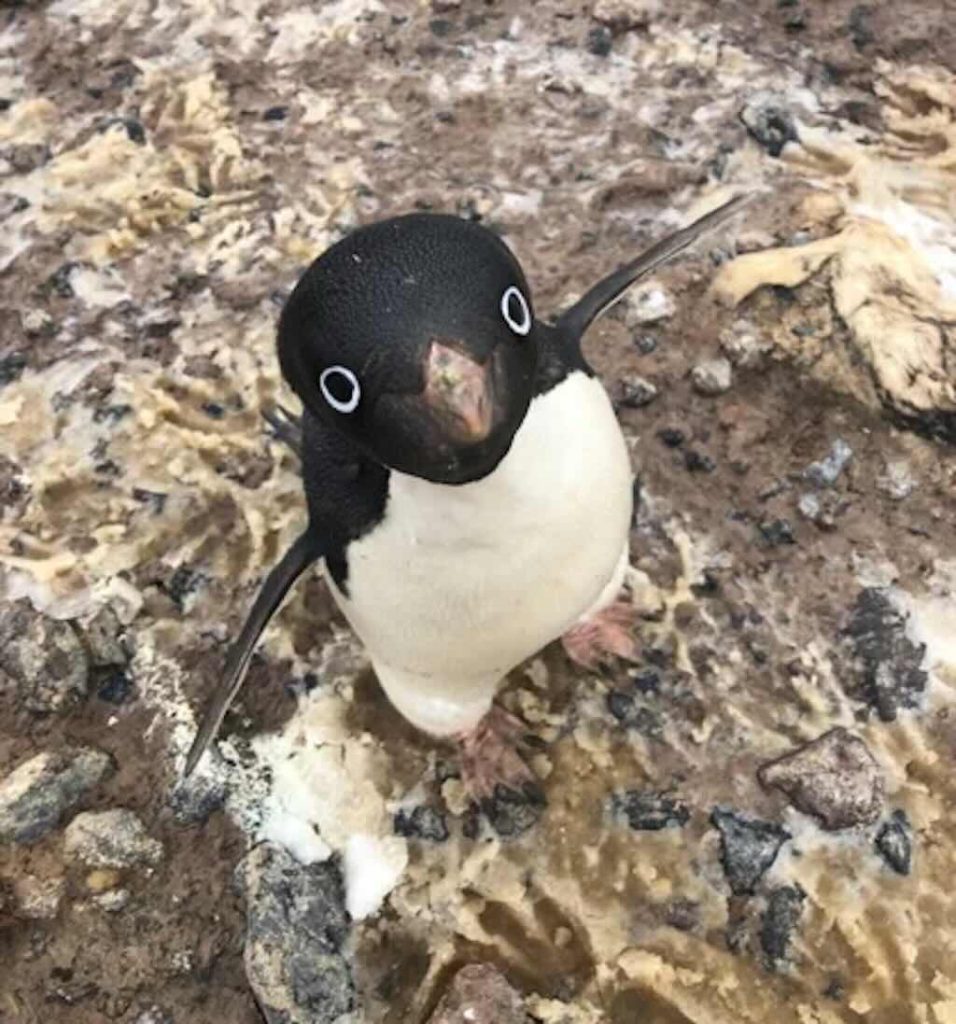
When visiting the Antarctic, you read all the time about how many penguins or seals are on one or other island or beach. But how do we ‘know’ this?
Let’s look at penguins as an example.
Our friend above is an actual photo taken with a drone.
Penguin population estimation is a complex process that involves a combination of direct and indirect survey methods, remote sensing technologies, and statistical modelling. What does that mean?
These are used in accessible colonies and serve as ground truth data for validating remote sensing estimates.
Direct nest or individual counts
- Point counts: Researchers count individuals in a defined area and extrapolate.
- Nest counts: Used for species that return to fixed nesting sites (e.g., Adélie and Emperor penguins).
- Photographic surveys: Taken from boats, ground, or drones (UAV – unmanned aerial vehicle) for later analysis.
- Scientists mark a subset of penguins (e.g., with leg bands or PIT tags) and later recapture a portion.
- The ratio of marked to unmarked individuals allows population estimation.
- The colony is divided into sections, and penguins are counted in a subset.
- Counts are scaled up using extrapolation models.
Remote sensing and aerial surveys
Used in large or inaccessible colonies, often via drones or satellites.
- Equipped with RGB and thermal cameras.
- Fly over colonies at specific altitudes to create detailed photomosaics.
- Image analysis software (e.g. OpenCV) detects penguins in images.
- High-resolution optical satellites (e.g. WorldView-3) detect penguins at 0.3–1.0m resolution.
- Synthetic Aperture Radar (SAR) can detect penguin movements and ice habitat changes.
- Guano Stain Mapping: Large-scale population estimation is done by detecting reddish-brown stains in snow and ice.
- Algorithms classify guano distribution in Sentinel-2 or Landsat-8 images.
- Models estimate population size based on average guano production per penguin.
Acoustic surveys (for Emperor Penguins)
Since Emperor penguins breed on sea ice, they are harder to detect.
- Acoustic sensors placed at colonies record penguin vocalisations.
- AI algorithms (e.g. CNNs or spectrogram analysis) identify individual calls and estimate colony size.
Statistical & Computational Modelling
- Observers count penguins at different distances.
- Detection probability function is applied to correct for unseen individuals.
- Integrate multiple data sources (ground counts, aerial surveys, and satellite data).
- Provide uncertainty estimates for population size.
- Convolutional Neural Networks (CNNs) detect and classify penguins in images.
- Supervised Learning: Models trained on manually labeled datasets.
- Unsupervised Learning: cluster analysis (e.g. k-means) for colony segmentation.
Challenges and sources of error
- Detection Bias: Penguins hidden by terrain or ice.
- Seasonal Variability: Populations change throughout the year.
- Image Resolution Limits: Low-resolution satellites may miscount penguins.
- Overlapping Guano Stains: May inflate population estimates.
Future developments
- Higher-resolution satellites (e.g., Pleiades Neo)
- Autonomous drone fleets for seasonal tracking
- AI-based acoustic monitoring networks
- Integration of environmental factors (e.g., climate models) for predictive forecasting
A Convolutional Neural Network (CNN) is a class of deep learning algorithms primarily used for processing structured grid data, such as images. CNNs are particularly effective in image recognition and classification tasks due to their ability to automatically and adaptively learn spatial hierarchies of features through back-propagation by using multiple building blocks, such as convolution layers, pooling layers, and fully connected layers.
And here is the answer to a question you probably didn’t ask, and probably don’t want to know. What is the average guano production per penguin?
It depends on the species, diet, colony size, and local environmental factors:-
- Emperor Penguin ~25–36 kg/year
- King Penguin ~18–29 kg/year
- Adélie Penguin ~11–22 kg/year
- Chinstrap Penguin ~15–26 kg/year
- Gentoo Penguin ~18–33 kg/year
And as a point of comparison, for humans its 36–91 kg/year (Western Diet – high in processed foods, lower fibre) and 91–182 kg/year (High-Fibre Diet – plant-based, traditional rural diets).
Field studies and datasets on penguin populations
This data was extracted from over 3,000 datasets that are held by the British Antarctic Survey, and this is just one example. Another example is the GBIF, the Global Biodiversity Information Facility, an international network and data infrastructure, which hosts over 110,000 datasets. And not forgetting the MAPPPD, the Mapping Application for Penguin Populations and Projected Dynamics, a one-stop shop for information on penguins in the Antarctic.
What is Krill?
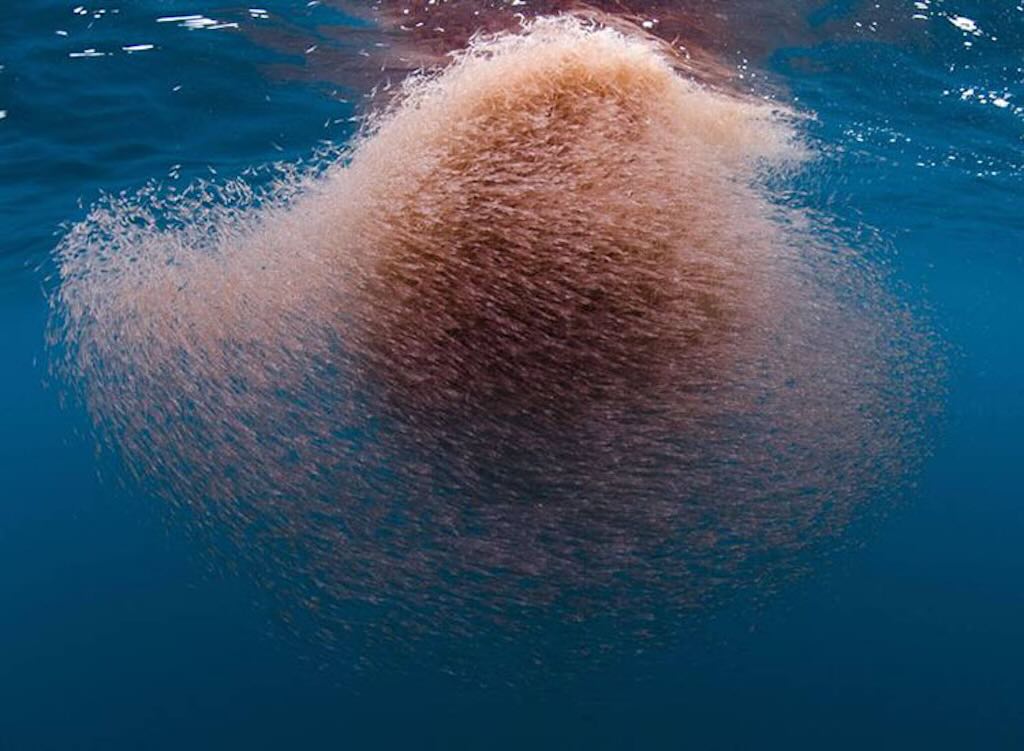
Birth and early development
The krill egg is neutrally buoyant at depths between 700 and 1000 meters, gradually sinking as it develops. Our example begins life as a fertilised egg in the Southern Ocean, approximately 50 km off the coast of South Georgia, an island in the subantarctic region. The water temperature at this depth is around -1.5°C to -1.8°C, near the freezing point of seawater due to its salinity (~34 ppt).
After about 10 days, our egg hatches into a nauplius larva, measuring just 0.6 mm in length and weighing approximately 0.002 mg. Lacking functional mouthparts, it survives on yolk reserves as it ascends toward the surface through convective currents and its own weak swimming movements. Over the next several weeks, it progresses through the metanauplius and calyptopis stages, eventually reaching the furcilia stage, where it develops functional feeding appendages and begins consuming phytoplankton.
This description is dependent upon the species of krill and the exact environmental conditions. For example, a hatching time of 10 days would be for a deep-water or cold-adapted species. Much depends upon the water temperature, at normal ocean temperatures (~0–2°C) eggs typically hatch within 3–6 days. And some krill species in even warmer waters may hatch in as little as 2–4 days.
Juvenile phase: growth and challenges
Now a juvenile krill of about 1 cm in length and weighing 10 mg, it resides in waters near South Georgia, where summer surface temperatures range from 0.5°C to 2°C. Its diet consists mainly of diatoms such as Fragilariopsis cylindrus and Thalassiosira antarctica. Our krill participates in diel vertical migration (DVM), staying at depths of 100-200 meters during the day to avoid predators such as lanternfish (Electrona antarctica) and surfacing at night to feed on phytoplankton.
During winter, food becomes scarce due to the seasonal retreat of sunlight, leading krill to adopt a more omnivorous diet, scraping microbial biofilms from ice and consuming copepods (Calanus propinquus) and detrital particles. Its metabolic rate slows down as temperatures drop to -1.8°C, and it can shrink in size to conserve energy. This reversible shrinkage is a unique adaptation among crustaceans, allowing survival in nutrient-poor conditions.
Adulthood and swarming
By age two, our krill reaches sexual maturity, measuring approximately 5 cm in length and weighing about 1 gram. Now part of a massive swarm, it moves in coordinated groups containing between 10,000 and 30,000 individuals per cubic meter. These swarms can stretch for several kilometers, sometimes containing billions of krill in a single aggregation, and serve as a critical food source for Antarctic predators, including Baleen whales (Balaenoptera bonaerensis), Adélie penguins (Pygoscelis adeliae), and Crabeater seals (Lobodon carcinophaga).
Krill’s reproductive cycle begins as daylight hours increase, triggering physiological changes. As a male, it develops spermatophores, which are transferred to a female via specialized thoracic appendages. Fertilisation occurs externally, and the female releases thousands of fertilised eggs (up to 10,000 per spawning event) into the depths. This ensures that the next generation continues, even as many adults succumb to predation.
A precarious existence: predators and climate change
Despite reaching maturity, krill faces constant threats. Leopard seals (Hydrurga leptonyx) patrol the ice edges, and myctophid fish hunt at mid-depths. However, the greatest challenge may come not from predators but from climate change. Rising ocean temperatures and diminishing sea ice threaten the krill’s habitat, reducing available winter food sources and disrupting population dynamics. Ocean acidification further complicates survival, potentially impairing shell development. The decrease in sea ice cover also affects the under-ice algae (Phaeocystis antarctica and Melosira arctica), a crucial food source during winter months.
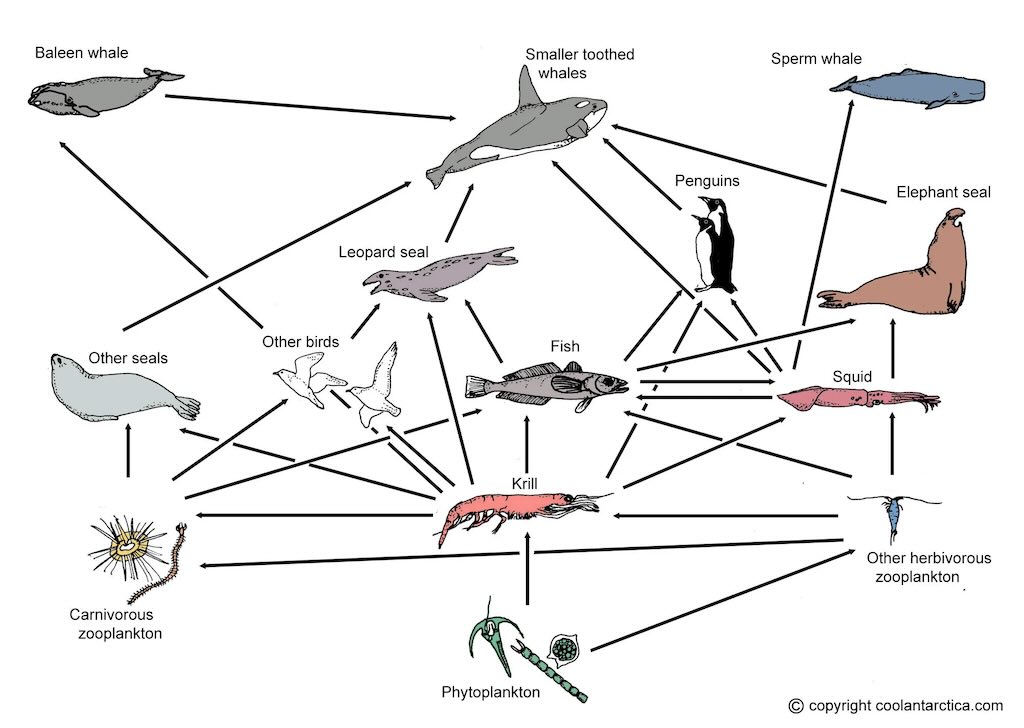
The end of the journey
At around six years of age, our krill reaches the twilight of its life. Growth slows, and carapace wear becomes evident. Finally, during a mid-winter feeding excursion, it is consumed by a juvenile Humpback whale (Megaptera novaeangliae). The whale filters thousands of krill in a single gulp, and krill biomass becomes part of the vast Antarctic marine food web, fueling the next trophic level.
In this cold and harsh yet immensely rich ecosystem, the life of krill is brief, perilous, yet ecologically vital. This illustrates the resilience and significance of Euphausia superba in the Southern Ocean.
Excursion - Kinnes Cove
The afternoon excursion was also later than usual, and was to Kinnes Cove (near Cape Kinnes), on Joinville Island, lying off the northeastern tip of the Antarctic Peninsula.
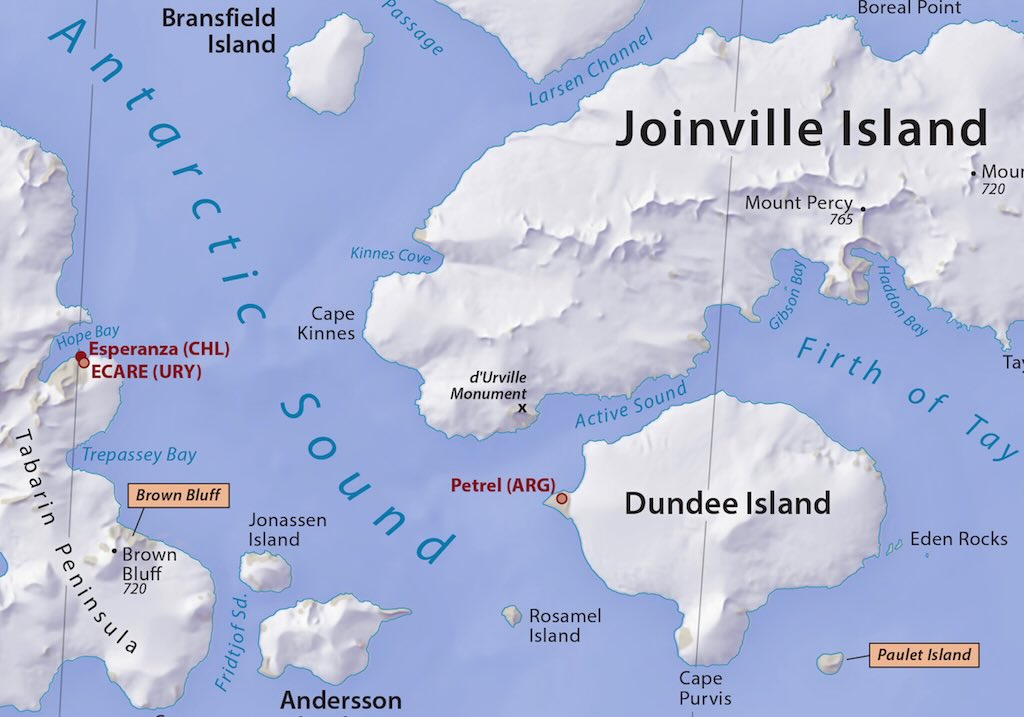
Kinnes Cove is a small inlet located on the western end of Joinville Island, off the northeastern tip of the Antarctic Peninsula. It lies between the Madder Cliffs and Cape Kinnes, forming part of the larger Suspiros Bay. The cove is characterised by steep, reddish rock cliffs rising approximately 305 meters from the sea, offering picturesque scenery with tabular icebergs and diverse wildlife.
The challenging terrain and harsh weather conditions have historically made anchoring difficult, which has influenced its naming history.
The naming of Kinnes Cove reflects its exploration history. During the Argentine Antarctic Expedition of 1951–52, Captain Emilio L. Díaz named the area “Bahía Suspiros“, translating to “Bay of Sighs,” due to the difficulties encountered while anchoring. The UK Antarctic Place-Names Committee later named it Kinnes Cove in 1957, in association with the nearby Cape Kinnes. Cape Kinnes was named by the Dundee Whaling Expedition (1892–93) after Robert Kinnes, a Dundee shipowner and merchant who sponsored the expedition.
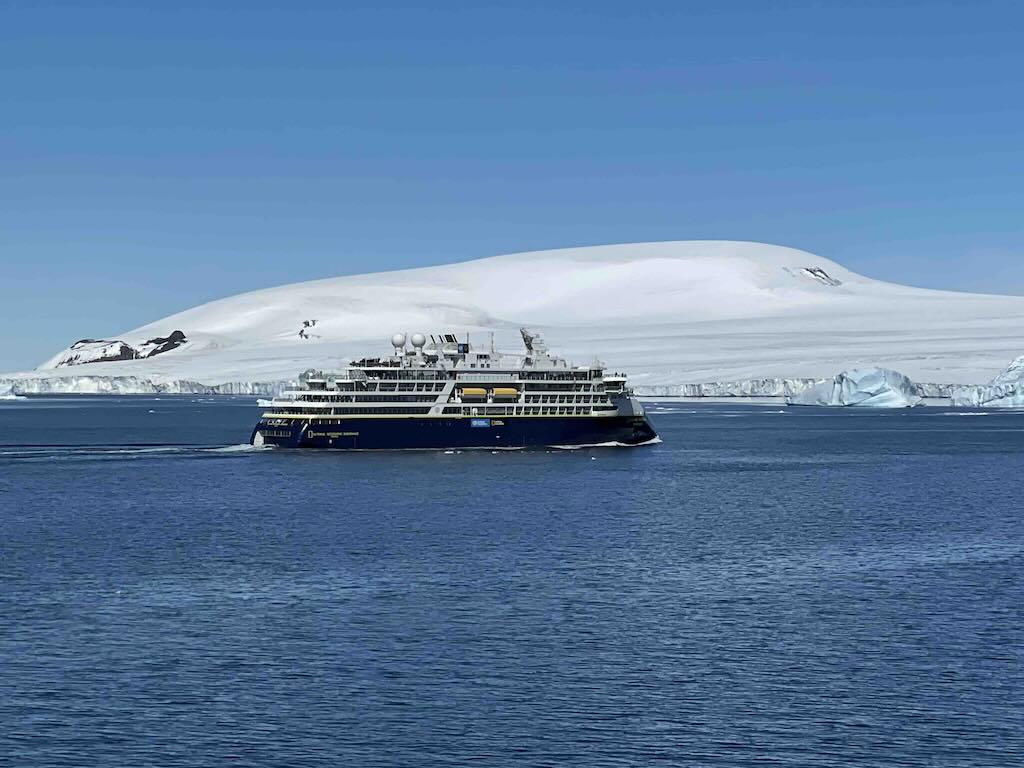
The first thing we discovered was that we were not alone. We found the National Geographic-Lindblad Expeditions Endurance, an ice-class launched in 2021, just leaving the cove.
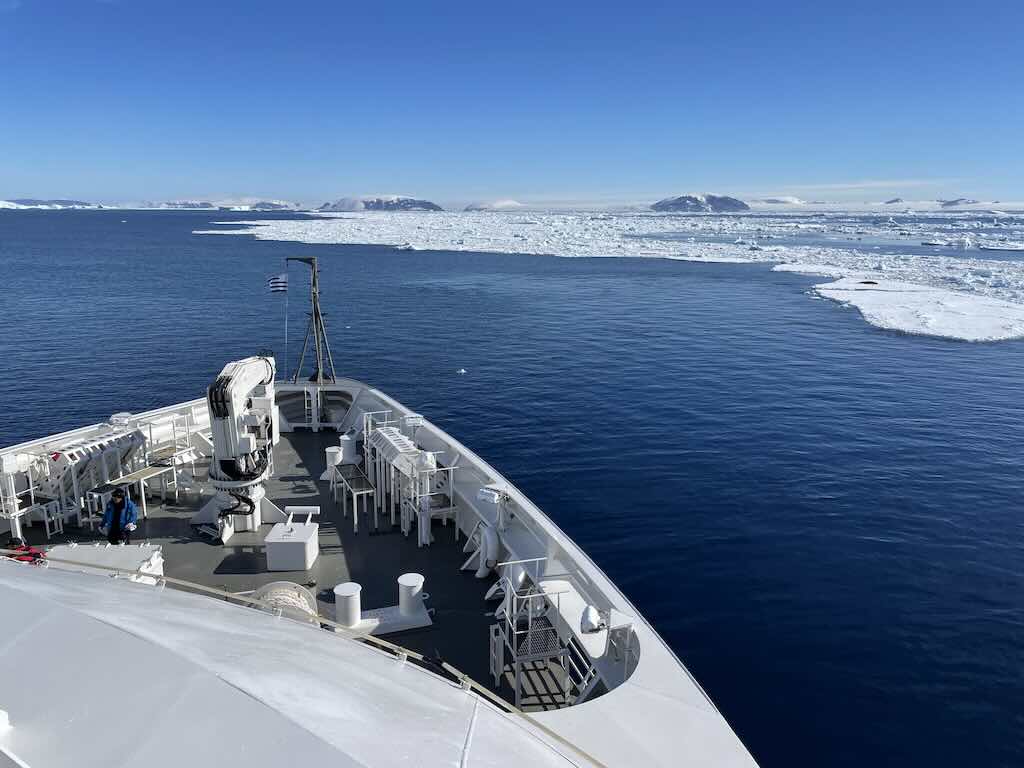
I didn’t go ashore this afternoon, preferring to relax and enjoy the scenery.
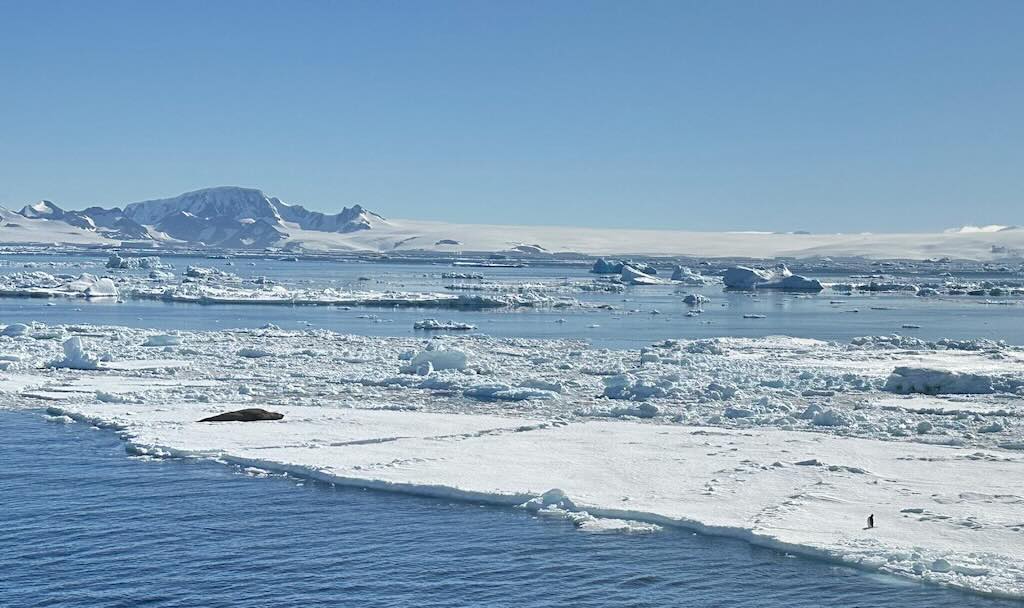
At 17:45 there was a daily recap & briefing, about what had happened during the day, and what was planned for the next day.
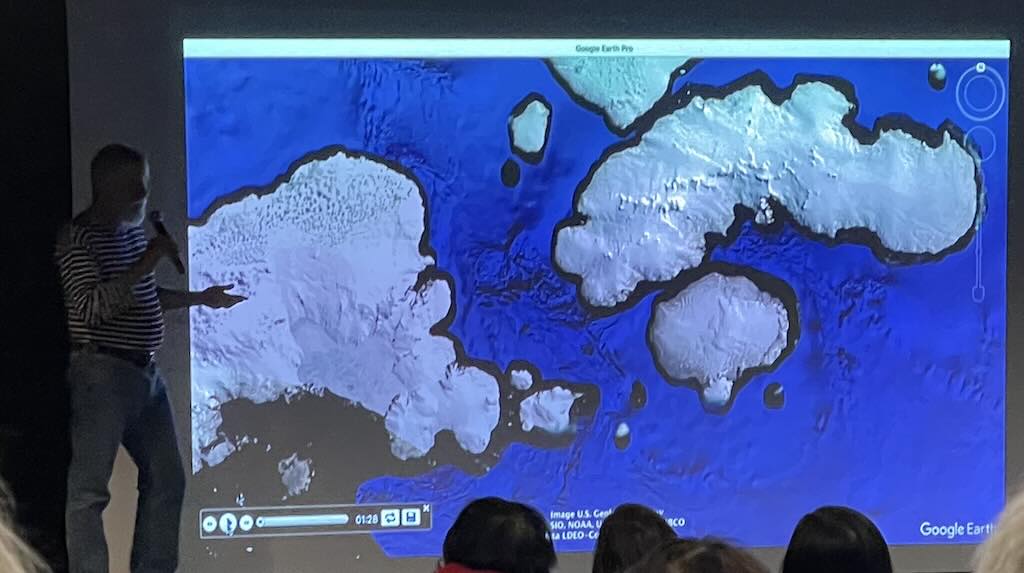
Can a Simple Stone Tell a Story?
I am a stone, and this is my story.
Birth in Fire
My existence began in a world of fire. Around 1 to 2 million years ago, deep beneath what is now called Brown Bluff on the Tabarin Peninsula, I was part of a magma chamber. The heat around me was unimaginable, over 1,000°C, perhaps even 1,300 °C. Convection currents within the Earth’s mantle pushed molten basaltic rock upward, and with a violent release of pressure, I was ejected as part of a volcanic eruption beneath a thick ice sheet.
As the magma contacted the ice and meltwater, it fragmented explosively. The surrounding environment was a phreatomagmatic battlefield, where magma and glacial meltwater interacted, creating tuff cones and hyaloclastites. In that eruption, my molten form rapidly cooled and solidified into volcanic rock, forming part of a pyroclastic deposit. The rapid cooling caused me to develop a fine-grained texture, with microscopic mineral crystals, primarily plagioclase, pyroxene, and olivine.
Entombed and Pressed
The eruption did not stop there. Over time, layers of volcanic ash, lapilli, and other pyroclasts accumulated above me. The ice sheet continued to exert tremendous pressure, compacting these deposits. I was locked in place, an immobile fragment in the geologic record, subjected to permafrost conditions. My surface darkened, oxidised, and fractured under the strain of thermal expansion and contraction.
The Ice’s Grip
For thousands of years, I remained trapped within the volcanic rock of Brown Bluff. The ice above thickened and thinned over glacial cycles, occasionally causing fracturing within the rock layers. The slow, imperceptible movement of ice, aided by freeze-thaw cycles, created fissures and cracks that weakened the matrix around me. This process, known as frost wedging, eventually loosened me from the rock mass.
One day, a large section of Brown Bluff sheared off due to mechanical weathering and ice melt-induced instability. This event dislodged me, and I tumbled down the slope, bouncing off other rocks, breaking into smaller pieces, and collecting a patina of scratches and gouges.
A Life on the Surface
For millennia, I have lain exposed on the surface of Brown Bluff, enduring some of the most extreme weather conditions on Earth. Katabatic winds howl over me, often exceeding 100 km/h, scouring my surface with fine volcanic dust and errant ice particles. In the summer months, when the Sun dips just enough to warm the black volcanic terrain, ice sublimates around me, exposing more of my surface.
The freeze-thaw cycles continue to shape me. Water from melting snow seeps into microscopic fractures within me, refreezing at night and expanding, slowly cleaving me apart. Lichen and extremophilic microorganisms colonise my surface, their bio-acidic secretions etching into my structure. These lifeforms, endolithic bacteria and lithophytic fungi, represent the only biological interactions I have known.
Encounters with Glacial Transport
On multiple occasions, seasonal meltwater has carried me a short distance downslope, further weathering my edges. Some of my fragments have even been incorporated into moraines, deposited by retreating glaciers. I have witnessed the ebb and flow of ice, the cycle of freeze and thaw, and the slow, persistent force of gravity pulling me ever downward.
Sharing Space with Others
I am not alone. Scattered across the landscape, my brethren take many forms. Some are basaltic like me, born of fire, while others are erratics, dragged from distant mountains by ancient glaciers. Together, we form the rugged terrain of Brown Bluff. Some of us are small, perfect for nesting birds, but I am too large to serve as a resting place for an Adélie penguin. I have seen my smaller counterparts become homes for nests, where eggs are laid and chicks are raised in the brief Antarctic summer. I do not envy them, nor do I fear such a fate. I am content in my place, a silent observer among my kin.
The World Around Me
Over time, I have observed the slow emergence of life around me. Hardy mosses and lichens cling to my surface, their green and orange hues a stark contrast to my dark volcanic form. In the summer months, I feel the faint tickle of Adélie penguins’ webbed feet as they traverse the rocky landscape, seeking nesting sites among my brethren. Occasionally, skuas circle overhead, their piercing cries breaking the silence of this frozen world. Weddell seals bask nearby on the ice, their heavy bodies barely disturbing the frozen ground beneath them. Each visitor is brief, their lives moving far faster than mine, yet I bear witness to their passage in my own slow, unchanging way.
Encounters with Humans
Not all visitors to Brown Bluff walk on flippers or feathers. Occasionally, I have seen humans, bundled in thick layers, their breath visible in the cold air. They tread carefully, their boots crunching the icy crust, their gloved hands reaching out to pick up stones like me. Some examine me, turning me over in their hands, inspecting my texture and weight before placing me back. Others carry tools, taking samples of my brethren for study, their voices echoing over the desolate landscape. Unlike the animals, humans do not migrate with the seasons. They come and go, leaving only footprints and the occasional metal marker in their wake. I am a silent observer of their brief passage, as I have been for all others before them.
A Stone’s Perception of Time and the Stars
Time moves differently for me than it does for the lifeforms around me. The life of a human, if I could measure such a thing, would be but a blink of an eye. I have seen glaciers advance and retreat, landforms shift, and mountains crumble, all in what would seem like mere moments to me. The flurry of activity from a nesting penguin, the brief exploration of a human scientist, the passing of a skua overhead, these all occur in what feels like a heartbeat. While life comes and goes, ephemeral as a gust of wind, I endure in stillness, unchanged for millennia, observing the world as it rushes past in a blur of fleeting moments.
And when the long Antarctic night falls, I am left to gaze upon the sky. The stars above, uncountable and ancient, are my only true companions in the vast stillness. Their cold light reaches me across the aeons, unchanged and steady, much like myself. If I could dream, perhaps my dreams would be of them, of drifting through the cosmos, untouched by time, watching galaxies spin as slowly as glaciers carve the land. But I do not dream, for I do not change. I simply am.
Today, I remain on the slopes of Brown Bluff, partially buried in volcanic debris and wind-blown sediment. I have lost some mass due to erosion, but I persist, my composition still revealing my fiery origins. I am an archive of Antarctica’s geologic and climatic history. A witness to volcanic activity, glacial movements, and the passage of time in one of the harshest environments on Earth.
What will become of me? Perhaps I will be carried to the sea by ice and meltwater, joining other volcanic fragments on the shores of the Weddell Sea. Perhaps I will continue to break down into finer particles, slowly becoming part of the Antarctic soil. But for now, I remain, as I have for millennia, a silent observer of the ever-changing Antarctic landscape.