Progress is Made: but Towards What?
Above is the Chicago Pile-1 (CP-1), the site of the first human-made, self-sustaining nuclear reaction. It consisted of a large, monolithic pile of uranium pellets and graphite blocks, with cadmium, indium, and silver control rods, but no radiation shield and cooling system. This is a rare photograph, but in fact it’s just one of the multiple builds and re-builds of the same pile.
By the time Fermi moved to Chicago I think he had a fairly clear idea what he was planning on doing. He was going to try and build an atomic ‘pile’. The fundamental idea of a ‘pile’ was a lattice structure with uranium concentrated in lumps regularly distributed in a matrix of (graphite) moderator. If the uranium and the moderator are mixed homogeneously, the neutrons on average will lose energy in small steps between passages through the uranium. So in the course of their reduction to thermal velocity the chance of passing through uranium at any given velocity, e.g. at a velocity corresponding to resonance absorption, is great. But, if the uranium is in large lumps spaced at large intervals in the moderator, the amounts of energy lost by neutrons between passages from one lump of uranium to another will be large and the chance of reaching a uranium lump with energy just equal to the energy of resonance absorption is relatively small. Thus the chance of absorption by uranium-238 to produce uranium-239, compared to the chance of absorption as thermal neutrons to cause fission, may be reduced sufficiently to allow a chain reaction to take place. If one knew the exact values of the cross-sections of each uranium isotope for each type of absorption and every range of neutron speed, and had similar knowledge for the moderator, one could calculate the “optimum lattice”, i.e. the best size, shape and spacing for the lumps of uranium in the matrix of moderator. Such data were only partially known, and as we have seen experiments were initiated at Columbia, and additional experiments were started at Princeton in February 1941. The key was to try and measure the absorption of neutrons by uranium under conditions similar to those expected in a chain-reacting pile employing graphite as moderator.
During 1939 and 1940 most of the work done on isotope separation and the chain reaction pile was performed in university laboratories by academic scientists funded primarily by private foundations. While the U.S. federal government began supporting uranium research in 1940, the pace appeared too leisurely to the scientific community and failed to convince scientists that their work was high priority. Certainly few were more inclined to this view than Ernest O. Lawrence, director of the Radiation Laboratory at the University of California in Berkeley. Lawrence was among those who thought that it was merely a matter of time before the United States was drawn into WW II, and he wanted the government to mobilise its scientific forces as rapidly as possible.
Specifically what Lawrence had on his mind in early 1941 were experiments taking place in his own laboratory using samples produced in the cyclotron. Studies on uranium fission fragments by Edwin Mattison McMillan and Philip Hauge Abelson led to the chemical identification of element 93, neptunium, while research by Glenn Theodore Seaborg revealed that an isotope of neptunium decayed to yet another transuranium (man-made) element. In February 1941, Seaborg identified this as element 94, which he later named plutonium (a natural follow-on based upon the names of the planets, after Neptune came Pluto).
By May 1941, Seaborg had proven that plutonium-239 was 1.7 times as likely as uranium-235 to fission. This finding made the Fermi-Szilárd experiment more important than ever as it suggested the possibility of producing large amounts of the fissionable plutonium in a uranium pile using plentiful uranium-238 and then separating the different elements chemically. Surely this would be less expensive and simpler than building isotope-separation plants. Lawrence, demonstrating his characteristic energy and impatience, launched a campaign to speed up uranium research. He began by proposing to convert his smaller cyclotron into a spectrograph to produce uranium-235. Since both the cyclotron and the spectrograph used a vacuum chamber and an electro-magnet, this conversion was relatively uncomplicated. Lawrence then took his case to Karl T. Compton and Alfred Lee Loomis at Harvard University, both doing radar work for the National Defense Research Committee. Infected by Lawrence’s enthusiasm, Compton forwarded Lawrence’s optimistic assessment on uranium research to Bush, warning that Germany was undoubtedly making progress and that Briggs and the Uranium Committee were moving too slowly. Compton also noted that the British were ahead of their American colleagues, even though, in his opinion, they were inferior in both numbers and ability.
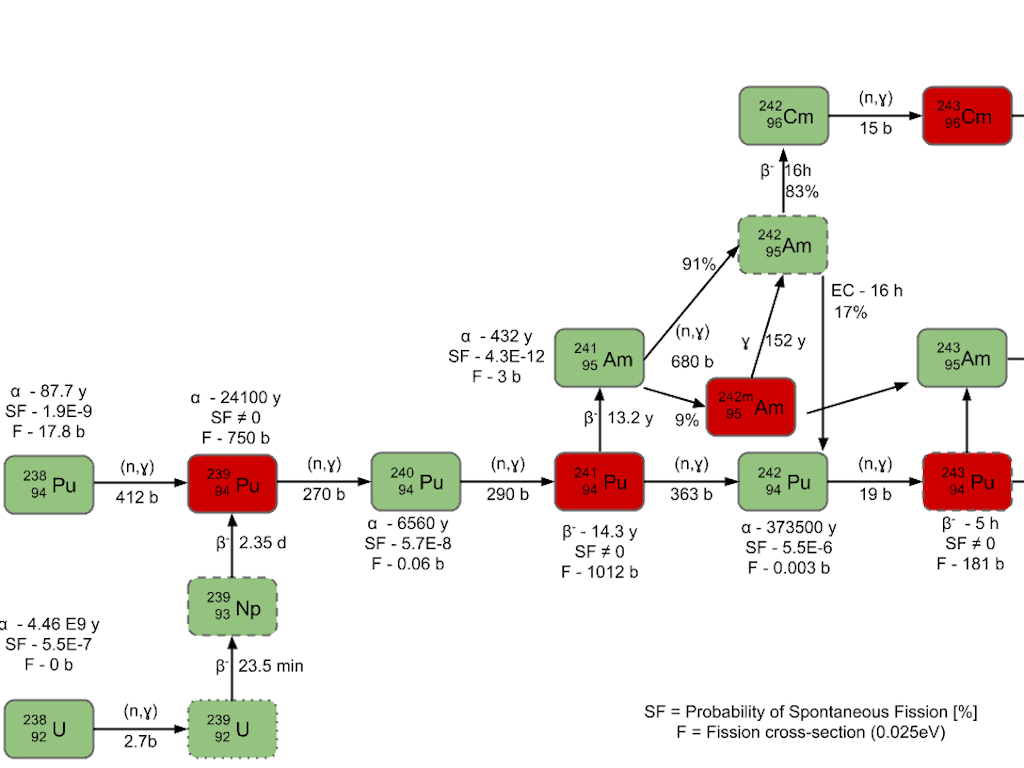
Chain of the transuranic elements. Neptunium (Np) was first synthesised by Edwin McMillan and Philip H. Abelson at the Berkeley Radiation Laboratory in 1940. Plutonium (Pu) was first produced and isolated in 1940 by Glenn T. Seaborg, Joseph W. Kennedy, Edwin M. McMillan, and Arthur C. Wahl. Americium (Am) was first produced in 1944 by the group of Glenn T. Seaborg from Berkeley, California, at the Metallurgical Laboratory of the University of Chicago, a part of the Manhattan Project. Curium (Cm) was first intentionally produced and identified in July 1944 by the group of Glenn T. Seaborg at the University of California, Berkeley. Other elements, such as Californium (Cf), were produced and identified after WW II.
Some books mention plutonium as being man-made, or discovered, or synthesised, but few mention plutonium-244, the only naturally occurring plutonium isotope, with a half-life of 80.8 million years. It has been postulated that this isotope has existed since the creation of Earth about 4.5 billion years ago (all heavy elements were formed in supernova explosions). It should also be mentioned that uranium in the Earth’s crust is also producing naturally plutonium, albeit on the order of one part in 1011 in pitchblende. And it is also worthwhile mentioning that apart from its formation in today’s nuclear reactors, plutonium was formed by the operation of naturally-occurring nuclear reactors in uranium deposits at Oklo in what is now Gabon, some two billion years ago. However, most of the plutonium in the Earth’s environment comes from weapons tests, which released about 3 tons of plutonium into the atmosphere. There exists also plutonium-245, plutonium-246, and plutonium-247, all with half-lives of a few days. In fact, plutonium has 23 known isotopes, ranging in mass from 228 to 247, and 9 isotopes have metastable states that usually last less than 1 second.
In discovering plutonium Seaborg’s team actually discovered (or made) two different ‘types’ of plutonium. They discovered that plutonium had two different oxidation states, i.e. in reacting with other elements plutonium forms two ions by giving up either 4 or 6 electrons, and each oxidation state has a completely different chemical behaviour. On top of that by treating plutonium with certain reagents the oxidation state will change. Immediately a possible ‘reduction-oxidation’ process (Redox) was imagined that could be used to purity plutonium. Initially plutonium in the lower oxidation state could be precipitated. Then it could be oxidised to the higher state and remain in solution during a second precipitation. Then it could be reduced to the lower oxidation state, and the same routine continued. The first samples of plutonium were microscopic, but Seaborg and his team managed to develop an industrial scale chemical process from scratch by August 18, 1942 (the scaling factor was nearly 1 to 1 billion). However, this feature was perhaps the least unusual of the characteristics that they would discover for plutonium.
Physicists might love plutonium, but it’s a chemists and engineers nightmare. They would discover that plutonium is in some ways just another metal, i.e. electro-positive, dissolves in mineral acids, but at the same time it’s a poor conductor. It has twice the density of iron, and is incredibly sensitive to temperature, and can undergo dramatic length changes (>20%). In one of the phases it will expand 5 times faster than iron, yet in another phase whilst being heated it will contract. Before turning liquid it goes through 6 different crystal structures and phase changes, including three expansions and two contractions (there is a 7th phase that appears at high pressures). It can change phases quite suddenly, and when it melts to become a liquid it contracts, becoming more dense. In its liquid state it has a very high surface tension, and it has the greatest viscosity of any element. Yet with the addition of a few atoms of aluminium or gallium, plutonium becomes malleable and easily pressed into different shapes. And just to make life interesting when in its close-packed crystal phase, it actually has its lowest density. And I have not mentioned plutonium’s chemical characteristics. Just as an example, plutonium acquires very rapidly a protective layer of plutonium oxide (like aluminium), and will corrode at a very, very slow rate. Except in moist air where it will corrode 200 times faster at room temperature and 100,000 times faster at 100°C. Plutonium ages by corrosion, but also from within by internal alpha-particle decay damage, and this damage makes plutonium noticeably warm to touch. And we should never forget that plutonium is pyrophoric above 500°C, but in powder form it can ignite at 150°C.
Americium-241, with a half-life of 432 years, was the first americium isotope to be isolated, and is still the one used today in many domestic smoke detectors, although they have been banned in most countries and have been replaced by smoke detectors using the photoelectric circuit.
In mid-2014 a plan was announced to extract americium-241 from the UK plutonium stockpile, much of it old. According to the U.K. National Nuclear Laboratory (NNL), about 250 kg of old civil plutonium (originally with about 10-14% plutonium-241) will yield 10 kg of americium-241. The European Space Agency is paying NNL to produce americium-241 for 10-watt (e) radioisotope thermoelectric generators (RTG’s) using very pure americium-241 recovered from old civil plutonium, as the isotope is much less expensive than plutonium-238 (now scarce). NASA has now decided to pay for the cost of domestic plutonium-238 processing, but the U.S. still has about 35 kg of plutonium-238 in reserve. Americium costs about $1500 per gram, whereas the U.S. stopped producing plutonium-238 in 1988 (and bought some 16.5 kg produced at Mayak) and the Russians have also stopped producing and selling it internationally. My understanding is that the Mars rover Curiosity was fuelled with 4.8 kg of plutonium-238, to produce 2 kW (th), the equivalent would have been around 15-20 kg of americium-241. Around 250 plutonium-powered pacemakers were manufactured, and 22 of them are still in service after 25 years (they were discontinued when it was found that they would not remain intact after cremation).
When Ernest O. Lawrence bombarding uranium with neutrons they discovered that the capture of fast neutrons by uranium-238 transmuted that isotope first into element-93 and then into element-94, which they named neptunium and plutonium, respectively. After further investigation of these transuranium elements, neither of which was then known to exist in nature, Lawrence’s group concluded that plutonium had the same fission characteristics as uranium-235, i.e. it could be split by neutrons and would, in turn, release more neutrons. Uranium-238, hitherto regarded as worthless for energy purposes, was in fact a prime source. Furthermore, as there was reason to believe that chemical separation of plutonium from uranium might prove more practicable than isotopic separation of uranium-235 from uranium-238, chances that an atomic bomb based on a fast neutron chain reaction could be built were tremendously increased.
In internal communications uranium-235 was often referred to as “25”, and plutonium as “49”, for the element no. 94, isotope atomic number 239.
The review committee requested by Vannevar Bush met in May, 1941 and submitted a report. On the basis of this report and the oral exposition by Briggs before a meeting of the NDRC, an appropriation of $267,000 was approved by the NDRC at its meeting of July 18, 1941, and the probability that much larger expenditures would be necessary was indicated. Bush asked for a second report with emphasis on engineering aspects, and in order to meet this request Oliver Ellsworth Buckley of the Bell Telephone Laboratories and Lewis Warrington Chubb of the Westinghouse Electrical and Manufacturing Company were added to the committee. The second report was submitted by Coolidge. As a result of new measurements of the fission cross-section of uranium-235 and with increasing conviction that isotope separation was possible, in September 1941, Compton and Lawrence suggested to J. B. Conant of NDRC, who was working closely with Bush, that a third report was desirable. Since Bush and Conant had learned during the summer of 1941 that the British also felt increasingly optimistic, the committee was asked to make another study of the whole subject. For this purpose the committee was enlarged by the addition of Warren Kendall Lewis, Robert Sanderson Mulliken, and George Bogdanovich Kistiakowsky. This third report was submitted by Compton on November 6, 1941.
George Bogdanovich Kistiakowsky (1900-1982) developed the explosives RDX and HMX, was responsible for developing shaped charges and explosive lenses used in the Manhattan Project weapons, and later served as Eisenhower’s scientific advisor.
The Atomic Bomb Moves to the Top of the List
The report of May 1941 mentioned first radioactive poisons (fission products), then atomic power (for ships and submarines), and finally atomic bombs, but the emphasis was on power generation. At this moment no one anticipated atomic explosives before 1945. However a report in July 1941 on engineering suggested that the uranium program should be supported because a self-sustaining reaction was bound to have tremendous impact. Isotope separation looked to be more challenging but it should continue to receive support. A demonstration of a chain reaction was called for as a separate project from the continued acquisition of fundamental data. Lawrence considered that both reports did not go far enough on the possibility of a bomb. However additional funding was allocated to work on the chain reaction.
Finally a report in November 1941 was specifically concerned with the “possibility of an explosive fission reaction with uranium-235”. This November report said “since our last report, the progress toward separation of the isotopes of uranium has been such as to make urgent a consideration of (1) the probability of success in the attempt to produce a fission bomb, (2) the destructive effect to be expected from such a bomb, (3) the anticipated time before its development can be completed and production be underway, and (4) the preliminary estimate of the costs involved“.
Bush and Lawrence met in New York City. Though he continued to support the Uranium Committee, Bush recognised that Lawrence’s assessment was not far off the mark. Bush shrewdly decided to appoint Lawrence as an advisor to Briggs, a move that quickly resulted in funding for plutonium work at Berkeley and for the mass spectrograph of Alfred Otto Carl Nier at Minnesota. Bush also asked the National Academy of Sciences to review the uranium research program. Headed by Arthur Compton of the University of Chicago and including Lawrence, this committee submitted its unanimous findings on May 17, 1941. Compton’s committee, however, failed to provide the practical-minded Bush with the evidence he needed that uranium research would pay off in the event the United States went to war in the near future. Compton’s group thought that increased uranium funding could produce radioactive material that could be dropped on an enemy by 1943, a pile that could power naval vessels in three or four years, and a bomb of enormous power at an indeterminate point, but certainly not before 1945. Compton’s report discussed bomb production only in connection with slow neutrons, a clear indication that much more scientific work remained to be done before an explosive device could be detonated.
Beginning in 1940 there was some interchange of information with the British and during the summer of 1941 Bush learned that they had been reviewing the whole subject in the period from April to July, 1941. They too had been interested in the possibility of using plutonium; in fact, a suggestion as to the advisability of investigating plutonium was contained in a letter from John Douglas Cockcroft to Ralph Howard Fowler dated December 28, 1940. Fowler, who was at that time acting as British scientific liaison officer in Washington, passed Cockcroft’s letter on to Lawrence. The British never pursued the plutonium possibility, since they felt their limited manpower should concentrate on uranium-235. Chadwick, at least, was convinced that a uranium-235 bomb of great destructive power could be made, and the whole British group felt that the separation of uranium-235 by diffusion was probably feasible.
Accounts of the British opinion, including the first draft of the British report reviewing the subject, were made available to Bush and Conant informally during the summer of 1941, although the official British report of July 15, 1941, was first transmitted to Conant by G. P. Thomson on October 3, 1941. Since, however, the British review was not made available to the committee of the National Academy of Sciences, the reports by the Academy committee and the British reports constituted independent evaluations of the prospects of producing atomic bombs.
The British report, prepared by a group codenamed the MAUD Committee (Committee for Military Application Uranium Detonation) and set up in spring 1940 to study the possibility of developing a nuclear weapon, maintained that a sufficiently purified critical mass of uranium-235 could fission even with fast neutrons. The British project was called “Tube Alloys”. Building upon theoretical work on atomic bombs performed by refugee physicists Rudolf Ernst Peierls and Otto Robert Frisch in 1940 and 1941, the MAUD report estimated that a critical mass of ten kilograms would be large enough to produce an enormous explosion. A bomb this size could be loaded on existing aircraft and be ready in approximately two years. Optimistically they had concluded that a uranium bomb could be built with an explosive power of 1,800 tons of TNT.
Americans had been in touch with the MAUD Committee since fall 1940, but it was the July 1941 MAUD report that helped the American bomb effort turn the corner. Here were specific plans for producing a bomb, produced by a distinguished group of scientists with high credibility in the United States, not only with Bush and Conant but with the President. The MAUD report dismissed plutonium production, thermal diffusion, the electro-magnetic method, and the centrifuge and called for gaseous-diffusion of uranium-235 on a massive scale. The British believed that uranium research could lead to the production of a bomb in time to affect the outcome of the war. While the MAUD report provided encouragement to Americans advocating a more extensive uranium research program, it also served as a sobering reminder that fission had been discovered in Nazi Germany almost three years earlier and that since spring 1940 a large part of the Kaiser Wilhelm Institute in Berlin had been set aside for uranium research.
For example, in Germany in April 1942, Baron Manfred von Ardenne already had completed an operational magnetic isotope separator in his laboratory in Berlin Lichterfelde, and his associate Friedrich Georg “Frtiz” Houtermans had correctly calculated the critical mass of uranium-235 the previous year. The MAUD Committee calculated the critical mass of uranium-235 to be 25 pounds (about 10 kg). Robert Oppenheimer himself, before joining the Manhattan Project, had estimated the critical mass at about 100 kilograms (220 pounds). His theoretical group at Berkeley quickly increased that by three times to 300 kilograms (660 pounds). As late as August 1943, when theorists at Los Alamos provided a critical mass estimate of 40 kilograms (88 pounds), the United States’ target number for enriched uranium production was still unknown.
Bush and Conant immediately went to work. After strengthening the Uranium Committee, particularly with the addition of Fermi as head of theoretical studies and Urey as head of isotope separation and heavy-water research (heavy-water was highly regarded as another neutron moderator), Bush asked yet another reconstituted National Academy of Sciences committee to evaluate the uranium program. This time he gave Compton specific instructions to address technical questions of critical mass and destructive capability, partially to verify the MAUD results.
In August 1943, the British were invited to join the Manhattan Project. This was the so-called Quebec Agreement signed by President Roosevelt and Winston Churchill. The British team included James Chadwick, Cyril Stanley Smith, Otto Frisch, Rudolf Peierls, and Emil Julius Klaus Fuchs (who later was convicted of spying for the Soviet Union).
Besides the official and semi-official conferences, there were many less formal discussions held, one of these being stimulated by Marcus Laurence Elwin Oliphant of England during his visit to the U.S. in the summer of 1941. The general conclusion was that the program should be pushed, and this conclusion in various forms was communicated to Bush by a number of people.
In the fall of 1941 Urey and Pegram were sent to England to get first-hand information on what was being done there. This was the first time that any Americans had been to England specifically in connection with the uranium problem. The report prepared by Urey and Pegram confirmed and extended the information that had been received previously.
As a result of the reports prepared by the National Academy committee, by the British, and by Urey and Pegram, and of the general urging by a number of physicists, Bush, as Director of the Office of Scientific Research and Development (of which NDRG was a part), decided that the uranium work should be pushed more aggressively.
Before the National Academy issued its third report and before Pegram and Urey visited England, Bush had taken up the whole uranium question with President Roosevelt and Vice-President Henry Agard Wallace. He summarised for them the British views, which were on the whole optimistic, and pointed out the uncertainties of the predictions. The President agreed that it was desirable to broaden the program, to provide a different organisation, to provide funds from a special source, and to open a complete interchange of information with the British. It was agreed to confine discussions of general policy to the following group: The President, Vice-President, Secretary of War, Chief of Staff, Bush, and Conant. This group was often referred to as the Top Policy Group.
The British knew that several kilograms of heavy-water a day were being produced in Norway, and that Germany had ordered considerable quantities of paraffin to be made using heavy hydrogen. It was difficult to imagine a use for these materials other than in work on the uranium problem. They feared that if the Germans got atomic bombs before the Allies did, the war might be over in a few weeks. The sense of urgency which Pegram and Urey brought back with them was of great importance.
By the time of submission of the National Academy’s third report and the return of Urey and Pegram from England, the general plan of the reorganisation was beginning to emerge. The Academy’s report was more conservative than the British report, as Bush pointed out in his letter of 27 November, 1941, to President Roosevelt. It was, however, sufficiently optimistic to give additional support to the plan of enlarging the work. The proposed reorganisation was announced at a meeting of the Uranium Section just before the Pearl Harbor attack (7 December, 1941).
It is odd that in some reports there is an explicit mentioned of a meeting that had already taken place on October 9, 1941, yet in other reports it is ignored or appears just as a footnote. The meeting took place between Roosevelt and Wallace and Vannevar Bush, and focussed on the atomic bomb, its potential, the cost of production, the risks including the German nuclear program, and even post-war control. The conclusion was to intensify, but not expand the program. Roosevelt understood that a great deal of money would be needed. This was not a meeting about making a bomb, but it gave Bush a free hand to find out if they could make a bomb. At the time the conclusions were limited to Roosevelt, Wallace, Bush, Conant, Henry Lewis Stimson (Secretary of War), and George Catlett Marshall (Chief of Staff).